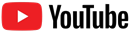
- TPC and eLearning
- What's NEW at TPC?
- Read Watch Interact
- Practice Review Test
- Teacher-Tools
- Request a Demo
- Get A Quote
- Subscription Selection
- Seat Calculator
- Ad Free Account
- Edit Profile Settings
- Metric Conversions Questions
- Metric System Questions
- Metric Estimation Questions
- Significant Digits Questions
- Proportional Reasoning
- Acceleration
- Distance-Displacement
- Dots and Graphs
- Graph That Motion
- Match That Graph
- Name That Motion
- Motion Diagrams
- Pos'n Time Graphs Numerical
- Pos'n Time Graphs Conceptual
- Up And Down - Questions
- Balanced vs. Unbalanced Forces
- Change of State
- Force and Motion
- Mass and Weight
- Match That Free-Body Diagram
- Net Force (and Acceleration) Ranking Tasks
- Newton's Second Law
- Normal Force Card Sort
- Recognizing Forces
- Air Resistance and Skydiving
- Solve It! with Newton's Second Law
- Which One Doesn't Belong?
- Component Addition Questions
- Head-to-Tail Vector Addition
- Projectile Mathematics
- Trajectory - Angle Launched Projectiles
- Trajectory - Horizontally Launched Projectiles
- Vector Addition
- Vector Direction
- Which One Doesn't Belong? Projectile Motion
- Forces in 2-Dimensions
- Being Impulsive About Momentum
- Explosions - Law Breakers
- Hit and Stick Collisions - Law Breakers
- Case Studies: Impulse and Force
- Impulse-Momentum Change Table
- Keeping Track of Momentum - Hit and Stick
- Keeping Track of Momentum - Hit and Bounce
- What's Up (and Down) with KE and PE?
- Energy Conservation Questions
- Energy Dissipation Questions
- Energy Ranking Tasks
- LOL Charts (a.k.a., Energy Bar Charts)
- Match That Bar Chart
- Words and Charts Questions
- Name That Energy
- Stepping Up with PE and KE Questions
- Case Studies - Circular Motion
- Circular Logic
- Forces and Free-Body Diagrams in Circular Motion
- Gravitational Field Strength
- Universal Gravitation
- Angular Position and Displacement
- Linear and Angular Velocity
- Angular Acceleration
- Rotational Inertia
- Balanced vs. Unbalanced Torques
- Getting a Handle on Torque
- Torque-ing About Rotation
- Properties of Matter
- Fluid Pressure
- Buoyant Force
- Sinking, Floating, and Hanging
- Pascal's Principle
- Flow Velocity
- Bernoulli's Principle
- Balloon Interactions
- Charge and Charging
- Charge Interactions
- Charging by Induction
- Conductors and Insulators
- Coulombs Law
- Electric Field
- Electric Field Intensity
- Polarization
- Case Studies: Electric Power
- Know Your Potential
- Light Bulb Anatomy
- I = ∆V/R Equations as a Guide to Thinking
- Parallel Circuits - ∆V = I•R Calculations
- Resistance Ranking Tasks
- Series Circuits - ∆V = I•R Calculations
- Series vs. Parallel Circuits
- Equivalent Resistance
- Period and Frequency of a Pendulum
- Pendulum Motion: Velocity and Force
- Energy of a Pendulum
- Period and Frequency of a Mass on a Spring
- Horizontal Springs: Velocity and Force
- Vertical Springs: Velocity and Force
- Energy of a Mass on a Spring
- Decibel Scale
- Frequency and Period
- Closed-End Air Columns
- Name That Harmonic: Strings
- Rocking the Boat
- Wave Basics
- Matching Pairs: Wave Characteristics
- Wave Interference
- Waves - Case Studies
- Color Addition and Subtraction
- Color Filters
- If This, Then That: Color Subtraction
- Light Intensity
- Color Pigments
- Converging Lenses
- Curved Mirror Images
- Law of Reflection
- Refraction and Lenses
- Total Internal Reflection
- Who Can See Who?
- Lab Equipment
- Lab Procedures
- Formulas and Atom Counting
- Atomic Models
- Bond Polarity
- Entropy Questions
- Cell Voltage Questions
- Heat of Formation Questions
- Reduction Potential Questions
- Oxidation States Questions
- Measuring the Quantity of Heat
- Hess's Law
- Oxidation-Reduction Questions
- Galvanic Cells Questions
- Thermal Stoichiometry
- Molecular Polarity
- Quantum Mechanics
- Balancing Chemical Equations
- Bronsted-Lowry Model of Acids and Bases
- Classification of Matter
- Collision Model of Reaction Rates
- Density Ranking Tasks
- Dissociation Reactions
- Complete Electron Configurations
- Elemental Measures
- Enthalpy Change Questions
- Equilibrium Concept
- Equilibrium Constant Expression
- Equilibrium Calculations - Questions
- Equilibrium ICE Table
- Intermolecular Forces Questions
- Ionic Bonding
- Lewis Electron Dot Structures
- Limiting Reactants
- Line Spectra Questions
- Mass Stoichiometry
- Measurement and Numbers
- Metals, Nonmetals, and Metalloids
- Metric Estimations
- Metric System
- Molarity Ranking Tasks
- Mole Conversions
- Name That Element
- Names to Formulas
- Names to Formulas 2
- Nuclear Decay
- Particles, Words, and Formulas
- Periodic Trends
- Precipitation Reactions and Net Ionic Equations
- Pressure Concepts
- Pressure-Temperature Gas Law
- Pressure-Volume Gas Law
- Chemical Reaction Types
- Significant Digits and Measurement
- States Of Matter Exercise
- Stoichiometry Law Breakers
- Stoichiometry - Math Relationships
- Subatomic Particles
- Spontaneity and Driving Forces
- Gibbs Free Energy
- Volume-Temperature Gas Law
- Acid-Base Properties
- Energy and Chemical Reactions
- Chemical and Physical Properties
- Valence Shell Electron Pair Repulsion Theory
- Writing Balanced Chemical Equations
- Mission CG1
- Mission CG10
- Mission CG2
- Mission CG3
- Mission CG4
- Mission CG5
- Mission CG6
- Mission CG7
- Mission CG8
- Mission CG9
- Mission EC1
- Mission EC10
- Mission EC11
- Mission EC12
- Mission EC2
- Mission EC3
- Mission EC4
- Mission EC5
- Mission EC6
- Mission EC7
- Mission EC8
- Mission EC9
- Mission RL1
- Mission RL2
- Mission RL3
- Mission RL4
- Mission RL5
- Mission RL6
- Mission KG7
- Mission RL8
- Mission KG9
- Mission RL10
- Mission RL11
- Mission RM1
- Mission RM2
- Mission RM3
- Mission RM4
- Mission RM5
- Mission RM6
- Mission RM8
- Mission RM10
- Mission LC1
- Mission RM11
- Mission LC2
- Mission LC3
- Mission LC4
- Mission LC5
- Mission LC6
- Mission LC8
- Mission SM1
- Mission SM2
- Mission SM3
- Mission SM4
- Mission SM5
- Mission SM6
- Mission SM8
- Mission SM10
- Mission KG10
- Mission SM11
- Mission KG2
- Mission KG3
- Mission KG4
- Mission KG5
- Mission KG6
- Mission KG8
- Mission KG11
- Mission F2D1
- Mission F2D2
- Mission F2D3
- Mission F2D4
- Mission F2D5
- Mission F2D6
- Mission KC1
- Mission KC2
- Mission KC3
- Mission KC4
- Mission KC5
- Mission KC6
- Mission KC7
- Mission KC8
- Mission AAA
- Mission SM9
- Mission LC7
- Mission LC9
- Mission NL1
- Mission NL2
- Mission NL3
- Mission NL4
- Mission NL5
- Mission NL6
- Mission NL7
- Mission NL8
- Mission NL9
- Mission NL10
- Mission NL11
- Mission NL12
- Mission MC1
- Mission MC10
- Mission MC2
- Mission MC3
- Mission MC4
- Mission MC5
- Mission MC6
- Mission MC7
- Mission MC8
- Mission MC9
- Mission RM7
- Mission RM9
- Mission RL7
- Mission RL9
- Mission SM7
- Mission SE1
- Mission SE10
- Mission SE11
- Mission SE12
- Mission SE2
- Mission SE3
- Mission SE4
- Mission SE5
- Mission SE6
- Mission SE7
- Mission SE8
- Mission SE9
- Mission VP1
- Mission VP10
- Mission VP2
- Mission VP3
- Mission VP4
- Mission VP5
- Mission VP6
- Mission VP7
- Mission VP8
- Mission VP9
- Mission WM1
- Mission WM2
- Mission WM3
- Mission WM4
- Mission WM5
- Mission WM6
- Mission WM7
- Mission WM8
- Mission WE1
- Mission WE10
- Mission WE2
- Mission WE3
- Mission WE4
- Mission WE5
- Mission WE6
- Mission WE7
- Mission WE8
- Mission WE9
- Vector Walk Interactive
- Name That Motion Interactive
- Kinematic Graphing 1 Concept Checker
- Kinematic Graphing 2 Concept Checker
- Graph That Motion Interactive
- Two Stage Rocket Interactive
- Rocket Sled Concept Checker
- Force Concept Checker
- Free-Body Diagrams Concept Checker
- Free-Body Diagrams The Sequel Concept Checker
- Skydiving Concept Checker
- Elevator Ride Concept Checker
- Vector Addition Concept Checker
- Vector Walk in Two Dimensions Interactive
- Name That Vector Interactive
- River Boat Simulator Concept Checker
- Projectile Simulator 2 Concept Checker
- Projectile Simulator 3 Concept Checker
- Hit the Target Interactive
- Turd the Target 1 Interactive
- Turd the Target 2 Interactive
- Balance It Interactive
- Go For The Gold Interactive
- Egg Drop Concept Checker
- Fish Catch Concept Checker
- Exploding Carts Concept Checker
- Collision Carts - Inelastic Collisions Concept Checker
- Its All Uphill Concept Checker
- Stopping Distance Concept Checker
- Chart That Motion Interactive
- Roller Coaster Model Concept Checker
- Uniform Circular Motion Concept Checker
- Horizontal Circle Simulation Concept Checker
- Vertical Circle Simulation Concept Checker
- Race Track Concept Checker
- Gravitational Fields Concept Checker
- Orbital Motion Concept Checker
- Angular Acceleration Concept Checker
- Balance Beam Concept Checker
- Torque Balancer Concept Checker
- Aluminum Can Polarization Concept Checker
- Charging Concept Checker
- Name That Charge Simulation
- Coulomb's Law Concept Checker
- Electric Field Lines Concept Checker
- Put the Charge in the Goal Concept Checker
- Circuit Builder Concept Checker (Series Circuits)
- Circuit Builder Concept Checker (Parallel Circuits)
- Circuit Builder Concept Checker (∆V-I-R)
- Circuit Builder Concept Checker (Voltage Drop)
- Equivalent Resistance Interactive
- Pendulum Motion Simulation Concept Checker
- Mass on a Spring Simulation Concept Checker
- Particle Wave Simulation Concept Checker
- Boundary Behavior Simulation Concept Checker
- Slinky Wave Simulator Concept Checker
- Simple Wave Simulator Concept Checker
- Wave Addition Simulation Concept Checker
- Standing Wave Maker Simulation Concept Checker
- Color Addition Concept Checker
- Painting With CMY Concept Checker
- Stage Lighting Concept Checker
- Filtering Away Concept Checker
- InterferencePatterns Concept Checker
- Young's Experiment Interactive
- Plane Mirror Images Interactive
- Who Can See Who Concept Checker
- Optics Bench (Mirrors) Concept Checker
- Name That Image (Mirrors) Interactive
- Refraction Concept Checker
- Total Internal Reflection Concept Checker
- Optics Bench (Lenses) Concept Checker
- Kinematics Preview
- Velocity Time Graphs Preview
- Moving Cart on an Inclined Plane Preview
- Stopping Distance Preview
- Cart, Bricks, and Bands Preview
- Fan Cart Study Preview
- Friction Preview
- Coffee Filter Lab Preview
- Friction, Speed, and Stopping Distance Preview
- Up and Down Preview
- Projectile Range Preview
- Ballistics Preview
- Juggling Preview
- Marshmallow Launcher Preview
- Air Bag Safety Preview
- Colliding Carts Preview
- Collisions Preview
- Engineering Safer Helmets Preview
- Push the Plow Preview
- Its All Uphill Preview
- Energy on an Incline Preview
- Modeling Roller Coasters Preview
- Hot Wheels Stopping Distance Preview
- Ball Bat Collision Preview
- Energy in Fields Preview
- Weightlessness Training Preview
- Roller Coaster Loops Preview
- Universal Gravitation Preview
- Keplers Laws Preview
- Kepler's Third Law Preview
- Charge Interactions Preview
- Sticky Tape Experiments Preview
- Wire Gauge Preview
- Voltage, Current, and Resistance Preview
- Light Bulb Resistance Preview
- Series and Parallel Circuits Preview
- Thermal Equilibrium Preview
- Linear Expansion Preview
- Heating Curves Preview
- Electricity and Magnetism - Part 1 Preview
- Electricity and Magnetism - Part 2 Preview
- Vibrating Mass on a Spring Preview
- Period of a Pendulum Preview
- Wave Speed Preview
- Slinky-Experiments Preview
- Standing Waves in a Rope Preview
- Sound as a Pressure Wave Preview
- DeciBel Scale Preview
- DeciBels, Phons, and Sones Preview
- Sound of Music Preview
- Shedding Light on Light Bulbs Preview
- Models of Light Preview
- Electromagnetic Radiation Preview
- Electromagnetic Spectrum Preview
- EM Wave Communication Preview
- Digitized Data Preview
- Light Intensity Preview
- Concave Mirrors Preview
- Object Image Relations Preview
- Snells Law Preview
- Reflection vs. Transmission Preview
- Magnification Lab Preview
- Reactivity Preview
- Ions and the Periodic Table Preview
- Periodic Trends Preview
- Chemical Reactions Preview
- Intermolecular Forces Preview
- Melting Points and Boiling Points Preview
- Bond Energy and Reactions Preview
- Reaction Rates Preview
- Ammonia Factory Preview
- Stoichiometry Preview
- Nuclear Chemistry Preview
- Gaining Teacher Access
- Task Tracker Directions
- Conceptual Physics Course
- On-Level Physics Course
- Honors Physics Course
- Chemistry Concept Builders
- All Chemistry Resources
- Users Voice
- Tasks and Classes
- Webinars and Trainings
- Subscription
- Subscription Locator
- 1-D Kinematics
- Newton's Laws
- Vectors - Motion and Forces in Two Dimensions
- Momentum and Its Conservation
- Work and Energy
- Circular Motion and Satellite Motion
- Thermal Physics
- Static Electricity
- Electric Circuits
- Vibrations and Waves
- Sound Waves and Music
- Light and Color
- Reflection and Mirrors
- Measurement and Calculations
- About the Physics Interactives
- Task Tracker
- Usage Policy
- Newtons Laws
- Vectors and Projectiles
- Forces in 2D
- Momentum and Collisions
- Circular and Satellite Motion
- Balance and Rotation
- Electromagnetism
- Waves and Sound
- Atomic Physics
- Forces in Two Dimensions
- Work, Energy, and Power
- Circular Motion and Gravitation
- Sound Waves
- 1-Dimensional Kinematics
- Circular, Satellite, and Rotational Motion
- Einstein's Theory of Special Relativity
- Waves, Sound and Light
- QuickTime Movies
- About the Concept Builders
- Pricing For Schools
- Directions for Version 2
- Measurement and Units
- Relationships and Graphs
- Rotation and Balance
- Vibrational Motion
- Reflection and Refraction
- Teacher Accounts
- Kinematic Concepts
- Kinematic Graphing
- Wave Motion
- Sound and Music
- About CalcPad
- 1D Kinematics
- Vectors and Forces in 2D
- Simple Harmonic Motion
- Rotational Kinematics
- Rotation and Torque
- Rotational Dynamics
- Electric Fields, Potential, and Capacitance
- Transient RC Circuits
- Light Waves
- Units and Measurement
- Stoichiometry
- Molarity and Solutions
- Thermal Chemistry
- Acids and Bases
- Kinetics and Equilibrium
- Solution Equilibria
- Oxidation-Reduction
- Nuclear Chemistry
- Newton's Laws of Motion
- Work and Energy Packet
- Static Electricity Review
- NGSS Alignments
- 1D-Kinematics
- Projectiles
- Circular Motion
- Magnetism and Electromagnetism
- Graphing Practice
- About the ACT
- ACT Preparation
- For Teachers
- Other Resources
- Solutions Guide
- Solutions Guide Digital Download
- Motion in One Dimension
- Work, Energy and Power
- Chemistry of Matter
- Names and Formulas
- Algebra Based On-Level Physics
- Honors Physics
- Conceptual Physics
- Other Tools
- Frequently Asked Questions
- Purchasing the Download
- Purchasing the Digital Download
- About the NGSS Corner
- NGSS Search
- Force and Motion DCIs - High School
- Energy DCIs - High School
- Wave Applications DCIs - High School
- Force and Motion PEs - High School
- Energy PEs - High School
- Wave Applications PEs - High School
- Crosscutting Concepts
- The Practices
- Physics Topics
- NGSS Corner: Activity List
- NGSS Corner: Infographics
- About the Toolkits
- Position-Velocity-Acceleration
- Position-Time Graphs
- Velocity-Time Graphs
- Newton's First Law
- Newton's Second Law
- Newton's Third Law
- Terminal Velocity
- Projectile Motion
- Forces in 2 Dimensions
- Impulse and Momentum Change
- Momentum Conservation
- Work-Energy Fundamentals
- Work-Energy Relationship
- Roller Coaster Physics
- Satellite Motion
- Electric Fields
- Circuit Concepts
- Series Circuits
- Parallel Circuits
- Describing-Waves
- Wave Behavior Toolkit
- Standing Wave Patterns
- Resonating Air Columns
- Wave Model of Light
- Plane Mirrors
- Curved Mirrors
- Teacher Guide
- Using Lab Notebooks
- Current Electricity
- Light Waves and Color
- Reflection and Ray Model of Light
- Refraction and Ray Model of Light
- Teacher Resources
- Subscriptions

- Newton's Laws
- Einstein's Theory of Special Relativity
- About Concept Checkers
- School Pricing
- Newton's Laws of Motion
- Newton's First Law
- Newton's Third Law
- Electric Potential Difference
- Electric Field and the Movement of Charge
- Electric Potential
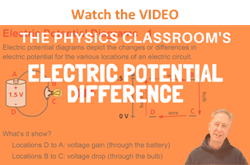

Electric Potential Difference and Simple Circuits
It is often convenient to speak of an electric circuit such as the simple circuit discussed here as having two parts - an internal circuit and an external circuit. The internal circuit is the part of the circuit where energy is being supplied to the charge. For the simple battery-powered circuit that we have been referring to, the portion of the circuit containing the electrochemical cells is the internal circuit. The external circuit is the part of the circuit where charge is moving outside the cells through the wires on its path from the high potential terminal to the low potential terminal. The movement of charge through the internal circuit requires energy since it is an uphill movement in a direction that is against the electric field . The movement of charge through the external circuit is natural since it is a movement in the direction of the electric field. When at the positive terminal of an electrochemical cell, a positive test charge is at a high electric pressure in the same manner that water at a water park is at a high water pressure after being pumped to the top of a water slide. Being under high electric pressure, a positive test charge spontaneously and naturally moves through the external circuit to the low pressure, low potential location.
As a positive test charge moves through the external circuit, it encounters a variety of types of circuit elements. Each circuit element serves as an energy-transforming device. Light bulbs, motors, and heating elements (such as in toasters and hair dryers) are examples of energy-transforming devices. In each of these devices, the electrical potential energy of the charge is transformed into other useful (and non-useful) forms. For instance, in a light bulb, the electric potential energy of the charge is transformed into light energy (a useful form) and thermal energy (a non-useful form). The moving charge is doing work upon the light bulb to produce two different forms of energy. By doing so, the moving charge is losing its electric potential energy. Upon leaving the circuit element, the charge is less energized. The location just prior to entering the light bulb (or any circuit element) is a high electric potential location; and the location just after leaving the light bulb (or any circuit element) is a low electric potential location. Referring to the diagram above, locations A and B are high potential locations and locations C and D are low potential locations. The loss in electric potential while passing through a circuit element is often referred to as a voltage drop . By the time that the positive test charge has returned to the negative terminal, it is at 0 volts and is ready to be re-energized and pumped back up to the high voltage, positive terminal.
Electric Potential Diagrams
An electric potential diagram is a convenient tool for representing the electric potential differences between various locations in an electric circuit. Two simple circuits and their corresponding electric potential diagrams are shown below.
In Circuit A, there is a 1.5-volt D-cell and a single light bulb. In Circuit B, there is a 6-volt battery (four 1.5-volt D-cells) and two light bulbs. In each case, the negative terminal of the battery is the 0 volt location. The positive terminal of the battery has an electric potential that is equal to the voltage rating of the battery. The battery energizes the charge to pump it from the low voltage terminal to the high voltage terminal. By so doing the battery establishes an electric potential difference across the two ends of the external circuit. Being under electric pressure , the charge will now move through the external circuit. As its electric potential energy is transformed into light energy and heat energy at the light bulb locations, the charge decreases its electric potential. The total voltage drop across the external circuit equals the battery voltage as the charge moves from the positive terminal back to 0 volts at the negative terminal. In the case of Circuit B, there are two voltage drops in the external circuit, one for each light bulb. While the amount of voltage drop in an individual bulb depends upon various factors (to be discussed later ), the cumulative amount of drop must equal the 6 volts gained when moving through the battery.
Investigate!
The electrical potential difference across the two inserts of a household electrical outlet varies with the country. Use the Household Voltages widget below to find out the household voltage values for various countries (e.g., United States, Canada, Japan, China, South Africa, etc.).
freestar.config.enabled_slots.push({ placementName: "physicsclassroom_incontent_3", slotId: "physicsclassroom_incontent_3" });
Check your understanding.
1. Moving an electron within an electric field would change the ____ the electron.
a. mass of b. amount of charge on c. potential energy of
Answer: C
When a force is required to move an electron in the direction of an electric field, its electrical potential energy increases. On the other hand, an electron moving opposite the direction of the electric field will decrease its electrical potential energy. This is because the electric field direction is in the direction which a positive charge spontaneously moves. An electron is negatively charged.
2. If an electrical circuit were analogous to a water circuit at a water park, then the battery voltage would be comparable to _____.
a. the rate at which water flows through the circuit b. the speed at which water flows through the circuit c. the distance that water flows through the circuit d. the water pressure between the top and bottom of the circuit e. the hindrance caused by obstacles in the path of the moving water
Answer: D
The battery establishes an electric potential difference across the two ends of the external circuit and thus causes the charge to flow. The battery voltage is the numerical value of this electric potential difference. In an analogous manner, it is the difference in water pressure between the top of the water slide and the bottom of the water slide that the water pump creates. This difference in water pressure causes water to flow down the slide. Because of the similarity between electric potential difference in an electric circuit and water pressure in a water park, the quantity electric potential difference is sometimes referred to as electric pressure.
3. If the electrical circuit in your Walkman were analogous to a water circuit at a water park, then the battery would be comparable to _____.
a. the people that slide from the elevated positions to the ground b. the obstacles that stand in the path of the moving water c. the pump that moves water from the ground to the elevated positions d. the pipes through which water flows e. the distance that water flows through the circuit
The electrochemical cells in an electric circuit supply the energy to pump the charge from the low energy terminal to the high energy terminal, thus providing a means by which the charge can flow. In an analogous manner, a water pump in a water park supplies the energy to pump the water from the low energy position to the high energy position. Because of the similarity between the battery in an electric circuit and a water pump in a water park, the battery is sometimes referred to as a charge pump.
4. Which of the following is true about the electrical circuit in your flashlight?
a. Charge moves around the circuit very fast - nearly as fast as the speed of light. b. The battery supplies the charge (electrons) that moves through the wires. c. The battery supplies the charge (protons) that moves through the wires. d. The charge becomes used up as it passes through the light bulb. e. The battery supplies energy that raises charge from low to high voltage. f. ... nonsense! None of these are true.
Answer: E
As emphasized on this page, the battery supplies the energy to move the charge through the battery, thus establishing and maintaining an electric potential difference. The battery does not supply electrons nor protons to the circuit; those are already present in the atoms of the conducting material. In fact, there would be no need to even supply charge at all since charge does not get used up in an electric circuit; only energy is used up in an electric circuit.
5. If a battery provides a high voltage, it can ____.
a. do a lot of work over the course of its lifetime b. do a lot of work on each charge it encounters c. push a lot of charge through a circuit d. last a long time
Answer: B
The electric potential difference or voltage of a battery is the potential energy difference across its terminals for every Coulomb of charge. A high voltage battery maximizes this ratio of energy/charge by doing a lot of work on each charge it encounters.
6. Compared to point D, point A is _____ electric potential.
a. 12 V higher in b. 12 V lower in c. exactly the same d. ... impossible to tell
Answer: A
The positive terminal of a battery is higher in electric potential than the negative terminal by an amount which is equal to the battery voltage.
7. The electric potential energy of a charge is zero at point _____.
Answer: D
The negative terminal of the battery is the low voltage location on a circuit. It is considered to be at 0 Volts.
8. Energy is required to force a positive test charge to move ___.
a. through the wire from point A to point B b. through the light bulb from point B to point C c. through the wire from point C to point D d. through the battery from point D to point A
Energy is required to cause a positive test charge to move against the electric field between the negative and the positive terminal.
9. The energy required to move +2 C of charge between points D and A is ____ J.
a. 0.167 b. 2.0 c. 6.0 d. 12 e. 24
A 12 volt battery would supply 12 Joules of electric potential energy per every 1 Coulomb of charge which moves between its negative and positive terminals. The ratio of the change in potential energy to charge is 12:1. Thus, 24 Joules would be the difference in potential energy for 2 Coulombs of charge.
10. The following circuit consists of a D-cell and a light bulb. Use >, <, and = symbols to compare the electric potential at A to B and at C to D. Indicate whether the devices add energy to or remove energy from the charge.
The electrochemical cell adds energy to the charge to move it from the low potential, negative terminal to the high potential, positive terminal. The light bulb removes energy from the charge. Thus, the charge is at lower energy and a lower electric potential when at locations C and A. Since there is no energy-consuming circuit element between locations B and D, these two locations have roughly the same electric potential. The same can be said of locations C and A.
11. Use your understanding of the mathematical relationship between work, potential energy, charge and electric potential difference to complete the following statements:
a. A 9-volt battery will increase the potential energy of 1 coulomb of charge by ____ joules. b. A 9-volt battery will increase the potential energy of 2 coulombs of charge by ____ joules. c. A 9-volt battery will increase the potential energy of 0.5 coulombs of charge by ____ joules. d. A ___-volt battery will increase the potential energy of 3 coulombs of charge by 18 joules. e. A ___-volt battery will increase the potential energy of 2 coulombs of charge by 3 joules. f. A 1.5-volt battery will increase the potential energy of ____ coulombs of charge by 0.75 joules. g. A 12-volt battery will increase the potential energy of ____ coulombs of charge by 6 joules.
This question targets your mathematical understanding of the relationship between the electrical potential difference, the voltage and the amount of charge. The relationship is expressed by the following equation:
b. A 9-Volt battery will increase the potential energy of 2 Coulombs of charge by 18 Joules.
c. A 9-Volt battery will increase the potential energy of 0.5 Coulombs of charge by 4.5 Joules.
d. A 6 -Volt battery will increase the potential energy of 3 Coulombs of charge by 18 Joules.
e. A 1.5 -Volt battery will increase the potential energy of 2 Coulombs of charge by 3 Joules.
f. A 1.5 Volt battery will increase the potential energy of 0.5 Coulombs of charge by 0.75 Joules.
g. A 12 Volt battery will increase the potential energy of 0.5 Coulombs of charge by 6 Joules.
- What is an Electric Circuit?
7.2 Electric Potential and Potential Difference
Learning objectives.
By the end of this section, you will be able to:
- Define electric potential, voltage, and potential difference
- Define the electron-volt
- Calculate electric potential and potential difference from potential energy and electric field
- Describe systems in which the electron-volt is a useful unit
- Apply conservation of energy to electric systems
Recall that earlier we defined electric field to be a quantity independent of the test charge in a given system, which would nonetheless allow us to calculate the force that would result on an arbitrary test charge. (The default assumption in the absence of other information is that the test charge is positive.) We briefly defined a field for gravity, but gravity is always attractive, whereas the electric force can be either attractive or repulsive. Therefore, although potential energy is perfectly adequate in a gravitational system, it is convenient to define a quantity that allows us to calculate the work on a charge independent of the magnitude of the charge. Calculating the work directly may be difficult, since W = F → · d → W = F → · d → and the direction and magnitude of F → F → can be complex for multiple charges, for odd-shaped objects, and along arbitrary paths. But we do know that because F → = q E → F → = q E → , the work, and hence Δ U , Δ U , is proportional to the test charge q . To have a physical quantity that is independent of test charge, we define electric potential V (or simply potential, since electric is understood) to be the potential energy per unit charge:
Electric Potential
The electric potential energy per unit charge is
Since U is proportional to q , the dependence on q cancels. Thus, V does not depend on q . The change in potential energy Δ U Δ U is crucial, so we are concerned with the difference in potential or potential difference Δ V Δ V between two points, where
Electric Potential Difference
The electric potential difference between points A and B , V B − V A , V B − V A , is defined to be the change in potential energy of a charge q moved from A to B , divided by the charge. Units of potential difference are joules per coulomb, given the name volt (V) after Alessandro Volta .
The familiar term voltage is the common name for electric potential difference. Keep in mind that whenever a voltage is quoted, it is understood to be the potential difference between two points. For example, every battery has two terminals, and its voltage is the potential difference between them. More fundamentally, the point you choose to be zero volts is arbitrary. This is analogous to the fact that gravitational potential energy has an arbitrary zero, such as sea level or perhaps a lecture hall floor. It is worthwhile to emphasize the distinction between potential difference and electrical potential energy.
Potential Difference and Electrical Potential Energy
The relationship between potential difference (or voltage) and electrical potential energy is given by
Voltage is not the same as energy. Voltage is the energy per unit charge. Thus, a motorcycle battery and a car battery can both have the same voltage (more precisely, the same potential difference between battery terminals), yet one stores much more energy than the other because Δ U = q Δ V . Δ U = q Δ V . The car battery can move more charge than the motorcycle battery, although both are 12-V batteries.
Example 7.4
Calculating energy.
Similarly, for the car battery, q = 60,000 C q = 60,000 C and
Significance
Check your understanding 7.4.
How much energy does a 1.5-V AAA battery have that can move 100 C?
Note that the energies calculated in the previous example are absolute values. The change in potential energy for the battery is negative, since it loses energy. These batteries, like many electrical systems, actually move negative charge—electrons in particular. The batteries repel electrons from their negative terminals ( A ) through whatever circuitry is involved and attract them to their positive terminals ( B ), as shown in Figure 7.12 . The change in potential is Δ V = V B − V A = + 12 V Δ V = V B − V A = + 12 V and the charge q is negative, so that Δ U = q Δ V Δ U = q Δ V is negative, meaning the potential energy of the battery has decreased when q has moved from A to B .
Example 7.5
How many electrons move through a headlight each second.
Entering the values for Δ U Δ U and Δ V Δ V , we get
The number of electrons n e n e is the total charge divided by the charge per electron. That is,
Check Your Understanding 7.5
How many electrons would go through a 24.0-W lamp each second from a 12-volt car battery?
The Electron-Volt
The energy per electron is very small in macroscopic situations like that in the previous example—a tiny fraction of a joule. But on a submicroscopic scale, such energy per particle (electron, proton, or ion) can be of great importance. For example, even a tiny fraction of a joule can be great enough for these particles to destroy organic molecules and harm living tissue. The particle may do its damage by direct collision, or it may create harmful X-rays, which can also inflict damage. It is useful to have an energy unit related to submicroscopic effects.
Figure 7.13 shows a situation related to the definition of such an energy unit. An electron is accelerated between two charged metal plates, as it might be in an old-model television tube or oscilloscope. The electron gains kinetic energy that is later converted into another form—light in the television tube, for example. (Note that in terms of energy, “downhill” for the electron is “uphill” for a positive charge.) Since energy is related to voltage by Δ U = q Δ V Δ U = q Δ V , we can think of the joule as a coulomb-volt.
Electron-Volt
On the submicroscopic scale, it is more convenient to define an energy unit called the electron-volt (eV), which is the energy given to a fundamental charge accelerated through a potential difference of 1 V. In equation form,
An electron accelerated through a potential difference of 1 V is given an energy of 1 eV. It follows that an electron accelerated through 50 V gains 50 eV. A potential difference of 100,000 V (100 kV) gives an electron an energy of 100,000 eV (100 keV), and so on. Similarly, an ion with a double positive charge accelerated through 100 V gains 200 eV of energy. These simple relationships between accelerating voltage and particle charges make the electron-volt a simple and convenient energy unit in such circumstances.
The electron-volt is commonly employed in submicroscopic processes—chemical valence energies and molecular and nuclear binding energies are among the quantities often expressed in electron-volts. For example, about 5 eV of energy is required to break up certain organic molecules. If a proton is accelerated from rest through a potential difference of 30 kV, it acquires an energy of 30 keV (30,000 eV) and can break up as many as 6000 of these molecules ( 30,000 eV ÷ 5 eV per molecule = 6000 molecules). ( 30,000 eV ÷ 5 eV per molecule = 6000 molecules). Nuclear decay energies are on the order of 1 MeV (1,000,000 eV) per event and can thus produce significant biological damage.
Conservation of Energy
The total energy of a system is conserved if there is no net addition (or subtraction) due to work or heat transfer. For conservative forces, such as the electrostatic force, conservation of energy states that mechanical energy is a constant.
Mechanical energy is the sum of the kinetic energy and potential energy of a system; that is, K + U = constant . K + U = constant . A loss of U for a charged particle becomes an increase in its K . Conservation of energy is stated in equation form as
where i and f stand for initial and final conditions. As we have found many times before, considering energy can give us insights and facilitate problem solving.
Example 7.6
Electrical potential energy converted into kinetic energy.
Entering the forms identified above, we obtain
We solve this for v :
Entering values for q , V , and m gives
Check Your Understanding 7.6
How would this example change with a positron? A positron is identical to an electron except the charge is positive.
Voltage and Electric Field
So far, we have explored the relationship between voltage and energy. Now we want to explore the relationship between voltage and electric field. We will start with the general case for a non-uniform E → E → field. Recall that our general formula for the potential energy of a test charge q at point P relative to reference point R is
When we substitute in the definition of electric field ( E → = F → / q ) , ( E → = F → / q ) , this becomes
Applying our definition of potential ( V = U / q ) ( V = U / q ) to this potential energy, we find that, in general,
From our previous discussion of the potential energy of a charge in an electric field, the result is independent of the path chosen, and hence we can pick the integral path that is most convenient.
Consider the special case of a positive point charge q at the origin. To calculate the potential caused by q at a distance r from the origin relative to a reference of 0 at infinity (recall that we did the same for potential energy), let P = r P = r and R = ∞ , R = ∞ , with d l → = d r → = r ^ d r d l → = d r → = r ^ d r and use E → = k q r 2 r ^ . E → = k q r 2 r ^ . When we evaluate the integral
for this system, we have
which simplifies to
This result,
is the standard form of the potential of a point charge. This will be explored further in the next section.
To examine another interesting special case, suppose a uniform electric field E → E → is produced by placing a potential difference (or voltage) Δ V Δ V across two parallel metal plates, labeled A and B ( Figure 7.14 ). Examining this situation will tell us what voltage is needed to produce a certain electric field strength. It will also reveal a more fundamental relationship between electric potential and electric field.
From a physicist’s point of view, either Δ V Δ V or E → E → can be used to describe any interaction between charges. However, Δ V Δ V is a scalar quantity and has no direction, whereas E → E → is a vector quantity, having both magnitude and direction. (Note that the magnitude of the electric field, a scalar quantity, is represented by E .) The relationship between Δ V Δ V and E → E → is revealed by calculating the work done by the electric force in moving a charge from point A to point B . But, as noted earlier, arbitrary charge distributions require calculus. We therefore look at a uniform electric field as an interesting special case.
The work done by the electric field in Figure 7.14 to move a positive charge q from A , the positive plate, higher potential, to B , the negative plate, lower potential, is
Work is W = F → · d → = F d cos θ W = F → · d → = F d cos θ ; here cos θ = 1 cos θ = 1 , since the path is parallel to the field. Thus, W = F d W = F d . Since F = q E F = q E , we see that W = q E d W = q E d .
Substituting this expression for work into the previous equation gives
The charge cancels, so we obtain for the voltage between points A and B
where d is the distance from A to B , or the distance between the plates in Figure 7.14 . Note that this equation implies that the units for electric field are volts per meter. We already know the units for electric field are newtons per coulomb; thus, the following relation among units is valid:
Furthermore, we may extend this to the integral form. Substituting Equation 7.6 into our definition for the potential difference between points A and B , we obtain
The potential difference is negative ( V is lower at B than at A ) when the displacement is in the same direction as the field. In other words, the electric field points toward lower electric potential. We are often only interested in the magnitude of the electric field, in which case you may see Δ V = E d Δ V = E d instead of | Δ V | = | E d | | Δ V | = | E d | and it is understood that all of the variables in the equation represent the magnitudes of the quantities. As a demonstration, from this we may calculate the potential difference between two points ( A and B ) equidistant from a point charge q at the origin, as shown in Figure 7.15 .
To do this, we integrate around an arc of the circle of constant radius r between A and B , which means we let d l → = r φ ^ d φ , d l → = r φ ^ d φ , while using E → = k q r 2 r ^ E → = k q r 2 r ^ . Thus,
for this system becomes
However, r ^ · φ ^ = 0 r ^ · φ ^ = 0 and therefore
This result, that there is no difference in potential along a constant radius from a point charge, will come in handy when we map potentials.
Example 7.7
What is the highest voltage possible between two plates.
Entering the given values for E and d gives
(The answer is quoted to only two digits, since the maximum field strength is approximate.)
Example 7.8
Field and force inside an electron gun.
- The expression for the magnitude of the electric field between two uniform metal plates is E = Δ V d . E = Δ V d . Since the electron is a single charge and is given 25.0 keV of energy, the potential difference must be 25.0 kV. Entering this value for V A B V A B and the plate separation of 0.0400 m, we obtain E = 25.0 kV 0.0400 m = 6.25 × 10 5 V/m . E = 25.0 kV 0.0400 m = 6.25 × 10 5 V/m .
- The magnitude of the force on a charge in an electric field is obtained from the equation F = q E . F = q E . Substituting known values gives F = ( 0.500 × 10 −6 C ) ( 6.25 × 10 5 V/m ) = 0.313 N . F = ( 0.500 × 10 −6 C ) ( 6.25 × 10 5 V/m ) = 0.313 N .

Example 7.9
Calculating potential of a point charge.
For the second step, V B − V A = − ∫ A B E → · d l → V B − V A = − ∫ A B E → · d l → becomes Δ V = − ∫ 0 24 ° k e q r 2 r ^ · r φ ^ d φ Δ V = − ∫ 0 24 ° k e q r 2 r ^ · r φ ^ d φ , but r ^ · φ ^ = 0 r ^ · φ ^ = 0 and therefore Δ V = 0 . Δ V = 0 . Adding the two parts together, we get 300 V.
Check Your Understanding 7.7
From the examples, how does the energy of a lightning strike vary with the height of the clouds from the ground? Consider the cloud-ground system to be two parallel plates.
Before presenting problems involving electrostatics, we suggest a problem-solving strategy to follow for this topic.
Problem-Solving Strategy
Electrostatics.
- Examine the situation to determine if static electricity is involved; this may concern separated stationary charges, the forces among them, and the electric fields they create.
- Identify the system of interest. This includes noting the number, locations, and types of charges involved.
- Identify exactly what needs to be determined in the problem (identify the unknowns). A written list is useful. Determine whether the Coulomb force is to be considered directly—if so, it may be useful to draw a free-body diagram, using electric field lines.
- Make a list of what is given or can be inferred from the problem as stated (identify the knowns). It is important to distinguish the Coulomb force F from the electric field E , for example.
- Solve the appropriate equation for the quantity to be determined (the unknown) or draw the field lines as requested.
- Examine the answer to see if it is reasonable: Does it make sense? Are units correct and the numbers involved reasonable?
This book may not be used in the training of large language models or otherwise be ingested into large language models or generative AI offerings without OpenStax's permission.
Want to cite, share, or modify this book? This book uses the Creative Commons Attribution License and you must attribute OpenStax.
Access for free at https://openstax.org/books/university-physics-volume-2/pages/1-introduction
- Authors: Samuel J. Ling, William Moebs, Jeff Sanny
- Publisher/website: OpenStax
- Book title: University Physics Volume 2
- Publication date: Oct 6, 2016
- Location: Houston, Texas
- Book URL: https://openstax.org/books/university-physics-volume-2/pages/1-introduction
- Section URL: https://openstax.org/books/university-physics-volume-2/pages/7-2-electric-potential-and-potential-difference
© Jul 23, 2024 OpenStax. Textbook content produced by OpenStax is licensed under a Creative Commons Attribution License . The OpenStax name, OpenStax logo, OpenStax book covers, OpenStax CNX name, and OpenStax CNX logo are not subject to the Creative Commons license and may not be reproduced without the prior and express written consent of Rice University.
Claimed by Sahil Arora (Fall 2016)
The Loop Rule, also known as Kirchhoff's Second Law, is a fundamental principle of electric circuits which states that the sum of potential differences around a closed circuit is equal to zero. More simply, when you travel around an entire circuit loop, you will return to the starting voltage. Note that is only true when the magnetic field is neither fluctuating nor time varying. If a changing magnetic field links the closed loop, then the principle of energy conservation does not apply to the electric field, causing the Loop Rule to be inaccurate in this scenario.
- 1.1 A Mathematical Model
- 1.2 A Computational Model
- 2.2 Middling
- 2.3 Difficult
- 3 Connectedness
- 5.1 Further Reading
- 5.2 External Links
- 6 References
The Main Idea
(The Energy Principle and the Loop Rule)
The loop rule simply states that in any round trip path in a circuit, Electric Potential equals zero. Keep in mind that this applies through ANY round trip path; there can be multiple round trip paths through more complex circuits. This principle deals with the conservation of energy within a circuit. Loop Rule and Node Rule are the two fundamental principles of electric circuits and are used to determine the behaviors of electric circuits. This principle is often used to solve for resistance or current passing through of light bulbs and other resistors, as well as the capacitance or charge of capacitors in a circuit.
A Visual Model
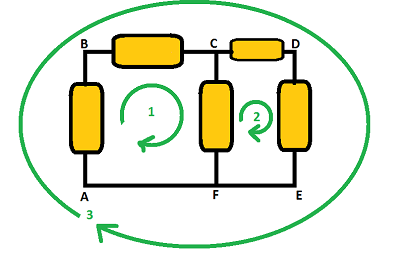
LOOP 1: [math]\displaystyle{ \Delta {V}_{AB} + \Delta {V}_{BC} + \Delta {V}_{CF} + \Delta {V}_{FA} = 0 }[/math]
LOOP 2: [math]\displaystyle{ \Delta {V}_{FC} + \Delta {V}_{CD} + \Delta {V}_{DE} + \Delta {V}_{EF} = 0 }[/math]
LOOP 3: [math]\displaystyle{ \Delta {V}_{AB} + \Delta {V}_{BC} + \Delta {V}_{CD} + \Delta {V}_{DE} + \Delta {V}_{EF} + \Delta {V}_{FA} = 0 }[/math]
To figure out the sign of the voltages, act as an observer walking along the path. Start at the negative end of the emf and continue walking along the path. The emf will be positive in the loop rule because you are moving from low to high voltage. Once you reach a resistor or capacitor, this will be negative in the loop rule equation because it is high to low voltage. Continue along the path until you return to the starting position.
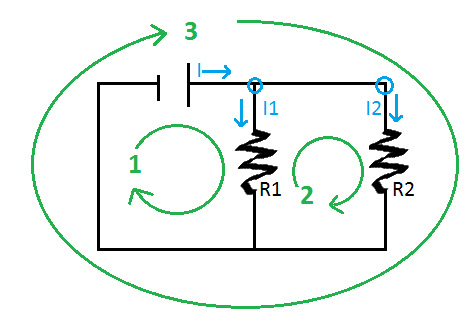
LOOP 1: [math]\displaystyle{ emf - I_1R_1 = 0 }[/math]
LOOP 2: [math]\displaystyle{ -I_1R_1 + I_2R_2 = 0 }[/math]
LOOP 3: [math]\displaystyle{ emf - I_2R_2 = 0 }[/math]
A Mathematical Model
A mathematical representation is:
where n is the number of voltages being measured in the loop, as well as
along any closed path in a circuit.
A Computational Model
This is a pretty cool model of how the Loop Rule is applied and calculated. You can change the direction of the current as well as the voltage of the batteries. To turn off the voice, press the Audio Tutorial button. To test your knowledge, click on the Concept Questions and Notes buttons, they have some questions and useful information in them.
This is an online circuit simulator. It opens up with an LRC circuit that has current running through it. The graphs on the bottom show the voltage as the current runs through the circuit. You can see that after a full loop the voltage is 0, verifying the loop rule. You can make all sorts of different circuits and loops and see for yourself.
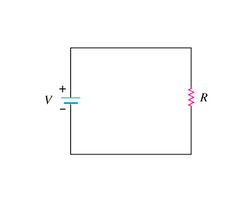
The circuit shown above consists of a single battery and a single resistor. The resistance of the wires is negligible for this problem.
If the [math]\displaystyle{ emf }[/math] is 5 V and the resistance of the resistor is 10 ohms, what is the current passing through the resistor?
Although we can solve this using the [math]\displaystyle{ V = IR }[/math] equation for the whole loop, let's examine this problem using the loop rule equation.
The loop rule equation would be [math]\displaystyle{ {V}_{battery} - {V}_{resistor} = 0 }[/math]
Since we know the [math]\displaystyle{ emf }[/math] of the battery we just need to find the potential difference through the resistor. For this we can use the equation of [math]\displaystyle{ V = IR }[/math] .
Thus we now have an loop rule equation of [math]\displaystyle{ emf - IR = 0 }[/math] From here it is a relatively simple process to find the current. We can rewrite the loop rule equation as [math]\displaystyle{ emf = IR }[/math] and then plug in 5 for the emf and 10 for the resistance, leaving us with I = .5 amperes.
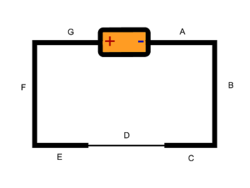
The circuit shown above consists of a single battery, whose [math]\displaystyle{ emf }[/math] is 1.3 V, and three wires made of the same material, but having different cross-sectional areas. Let the length of the thin wires be [math]\displaystyle{ {L}_{thick} }[/math] and the length of the thin wire be [math]\displaystyle{ {L}_{thin} }[/math] Find a loop rule equation that starts at the negative end of the battery and goes counterclockwise through the circuit.
When beginning this problem, you must notice that the difference in cross-sectional areas affects the electric field in each wire. Because of this we will denote the electric field at D. as [math]\displaystyle{ {E}_{D} }[/math] and the electric field everywhere else as [math]\displaystyle{ {E}_{A} }[/math] . To begin we will go around the circuit clockwise and add up each component. First, we know that the [math]\displaystyle{ emf }[/math] of the battery is 1.3 V. Then, we will add up the potential voltage of each of the wires.
Remember that the electric potential of a wire is equal to the product of the electric field and the length of the wire. From this we can now find the potential difference of each section of the wires. The electric potential of location A - C is [math]\displaystyle{ {E}_{A} * {L}_{thick} }[/math] . This is the same for the electric potential of locations E - G of the wire. For the thin section of the wire, the electric potential is [math]\displaystyle{ {E}_{D} * {L}_{thin} }[/math] . From here we just go around the circuit counterclockwise and add each potential difference to the loop rule equation.
Thus we can find that a loop rule equation is: [math]\displaystyle{ emf - 2 ({E}_{A} * {L}_{thick}) - {E}_{D} * {L}_{thin} = 0 }[/math]
This can also be rewritten as: [math]\displaystyle{ emf = 2 ({E}_{A} * {L}_{thick}) + {E}_{D} * {L}_{thin} = 0 }[/math]
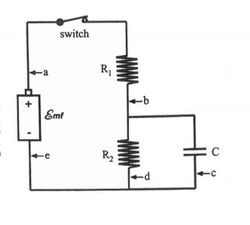
For the circuit above, imagine a situation where the switch has been closed for a long time. Calculate the current at a,b,c,d,e and charge Q of the capacitor. Answer these using [math]\displaystyle{ emf, {R}_{1}, {R}_{2}, and \space C }[/math]
First, write loop rule equations for each of the possible loops in the circuit. There are 3 loop equations that are possible.
[math]\displaystyle{ emf - {I}_{1}{R}_{1} - {I}_{2}{R}_{2} = 0 }[/math]
[math]\displaystyle{ emf - {I}_{1}{R}_{1} - Q/C = 0 }[/math]
[math]\displaystyle{ {I}_{2}{R}_{2} - Q/C = 0 }[/math]
From here, we can then solve for the current passing through a,b,d and e. We also know that the current passing through these points must be the same so [math]\displaystyle{ {I}_{1} = {I}_{2} }[/math]
[math]\displaystyle{ emf - {I}_{1}{R}_{1} - {I}_{1}{R}_{2} = 0 }[/math]
[math]\displaystyle{ emf = {I}_{1}({R}_{1} + {R}_{2}) = 0 }[/math]
[math]\displaystyle{ emf/({R}_{1} + {R}_{2}) = {I}_{1} }[/math]
So the current at a,b,d,e = [math]\displaystyle{ emf/({R}_{1} + {R}_{2}) }[/math]
You must also know that once a capacitor is charging for a long time, current no longer flows through the capacitor. We can then easily solve for c because since current is no longer flowing through the capacitor, the current at c = 0.
Lastly, we will use the loop rule equation of [math]\displaystyle{ {I}_{2}{R}_{2} - Q/C = 0 }[/math] to solve for Q.
[math]\displaystyle{ {I}_{2}{R}_{2} = Q/C }[/math]
[math]\displaystyle{ C*({I}_{2}{R}_{2}) = Q }[/math]
Since [math]\displaystyle{ {I}_{1} = {I}_{2} }[/math] , [math]\displaystyle{ C*({I}_{1}{R}_{2}) = Q }[/math]
We can plug in what we found [math]\displaystyle{ {I}_{1} }[/math] equals from before.
[math]\displaystyle{ C* (emf/({R}_{1} + {R}_{2})){R}_{2}) = Q }[/math]
Lastly, Q = [math]\displaystyle{ C* (emf/({R}_{1} + {R}_{2})){R}_{2} }[/math] and we have now solved the problem.
Current at a,b,d,e = [math]\displaystyle{ emf/({R}_{1} + {R}_{2}) }[/math]
Current at c = 0
Q = [math]\displaystyle{ C* (emf/({R}_{1} + {R}_{2})){R}_{2} }[/math]
Connectedness
The Loop Rule is simply an extension of the conservation of energy applied to circuits. Circuits are ubiquitous as they are featured in almost every technology today. Our understanding of these technologies is rooted in the empirical discoveries made in the mid 19th century, and further boosted by the the advancement of theoretical knowledge due to the likes of Faraday and Maxwell.
One interesting note about Loop Rule is that is does not apply universally to all circuits. In particular, AC (alternating current) circuits at high frequencies, have a fluctuating electric charge that changes direction. This causes the electric potential of a round trip path around the circuit to no longer be zero. However, DC (direct current) circuits, and low frequency circuits in general, still follow the loop rule.
The Loop Rule is formally known as the Kirchhoff Voltage Laws , named after Gustav Kirchhoff , the scientist who discovered and defined this fundamental concept of electric circuits. He discovered this during his time as a student at Albertus University of Königsberg in 1845. Kirchoff went on to explore the topics of spectroscopy and black body radiation after his graduation from Albertus. Nowadays, it is used very often in electrical engineering.
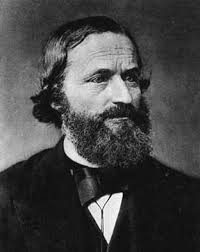
Further Reading
Other Circuit Concepts you can check out :
Resistors and Conductivity
Kirchoff's Circuit Laws - Wikipedia
External Links
If you want to test your knowledge, Khan Academy's DC Circuit Analysis under the Electrical Engineering Topic is an excellent resource. There are questions, videos, and written explanations to help you understand not just the loop rule, but the node rule as well. Click here to access it.
Loop Rule - Boundless.com Textbook
Doc Physics Video Lecture
Doc Physics Worked Example
Bozeman Science Lecture
- Dr. Nicholas Darnton's lecture notes
- https://en.wikipedia.org/wiki/Kirchhoff%27s_circuit_laws#Kirchhoff.27s_voltage_law
- https://www.khanacademy.org/science/electrical-engineering/ee-circuit-analysis-topic/ee-dc-circuit-analysis
- Chabay, Ruth W. Matter and Interactions: Electric and Magnetic Interactions. John Wiley, 2015. Print.
- http://www.ux1.eiu.edu/~cfadd/1360/28DC/Loop.html
- http://higheredbcs.wiley.com/legacy/college/cutnell/0470223553/concept_sims/sim34/sim34.html
- http://www.falstad.com/circuit/
- Schuster, D. (Producer). (2013). Kirchhoff's Loop and Junction Rules Theory [Motion picture]. United States of America: YouTube.
- Schuster, D. (Producer). (2013). Kirchhoff's Rules (Laws) Worked Example [Motion picture]. United States of America: YouTube.
- Anderson, P. (Producer). (2015). Kirchoff's Loop Rule [Motion picture]. United States of America: YouTube.
- Electric Fields and energy in circuits
Navigation menu
- Credit cards
- View all credit cards
- Banking guide
- Loans guide
- Insurance guide
- Personal finance
- View all personal finance
- Small business
- Small business guide
- View all taxes
You’re our first priority. Every time.
We believe everyone should be able to make financial decisions with confidence. And while our site doesn’t feature every company or financial product available on the market, we’re proud that the guidance we offer, the information we provide and the tools we create are objective, independent, straightforward — and free.
So how do we make money? Our partners compensate us. This may influence which products we review and write about (and where those products appear on the site), but it in no way affects our recommendations or advice, which are grounded in thousands of hours of research. Our partners cannot pay us to guarantee favorable reviews of their products or services. Here is a list of our partners .
One-Way vs. Round-Trip Flights: When Is It Worth Booking Separately?
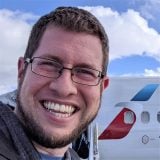
Many, or all, of the products featured on this page are from our advertising partners who compensate us when you take certain actions on our website or click to take an action on their website. However, this does not influence our evaluations. Our opinions are our own. Here is a list of our partners and here's how we make money .
Table of Contents
What is a round-trip flight?
What is a one-way flight, when booking cash tickets, when redeeming points and miles, consider booking open-jaw flights.
Are round-trip flights cheaper? The short answer is that it depends . Where you’re traveling to and if you’re paying with cash or miles are the biggest factors in the equation.
For instance, if you’re traveling between the U.S. and Europe and paying in cash, it's cheaper to book a round-trip flight. But if you’re paying for that same flight with miles, the price of two one-way tickets will most often be the same as a round-trip flight (though the taxes paid may vary).
There are other considerations besides just price when comparing round-trip versus one-way flights. Travelers might be surprised to find that booking a round-trip award flight could lead to issues if you have a change in plans mid-trip.
Here's a look at options to weigh when booking round-trip versus one-way flights and answers to some frequently asked questions.
» Learn more: The best travel credit cards right now
When you book a round-trip flight, it means you travel from Destination A to Destination B and you return back to Destination A from Destination B. Both flights are booked as one ticket.
So when you’re purchasing the ticket, you select your origin, destination, departure and return date. You make one payment, whether it's with cash or miles.
» Learn more: How to book a flight
A one-way flight, in contrast, is a ticket booked with no return. So you’re only booking a flight from Destination A to Destination B. You can purchase a second, separate ticket from Destination B back to Destination A (should you so choose). One-way flights can be booked with cash or miles.
These types of flights are a good option for those who don’t know their return date, are only traveling in one direction or booking an open-jaw itinerary .
» Learn more: The best days to book a flight and when to fly
When booking flights paid in cash, our recommendation is pretty simple: Book the option that's cheapest for your trip. If a round-trip flight is cheaper than two one-way flights, there's no reason to book two one-way flights.
If two one-way flights are the same price as a round-trip, it might make sense to book the one-way flight (as long as you don’t mind keeping track of the two separate reservations). Even though many airlines have eliminated change fees , it's much easier to change — or even cancel — a flight when there’s only one flight on the reservation to make a change to.
If you’re flying domestically in the U.S., the cost of two one-way flights will usually be the same as a round trip; however, when flying internationally, it can be much more expensive to book two one-way tickets.
Sample flight within the U.S.
In this example flight search on American Airlines , booking two one-way tickets from Newark to Miami will cost $154 for the outgoing flight and $124 for the return, for a total of $278.
Outgoing flight
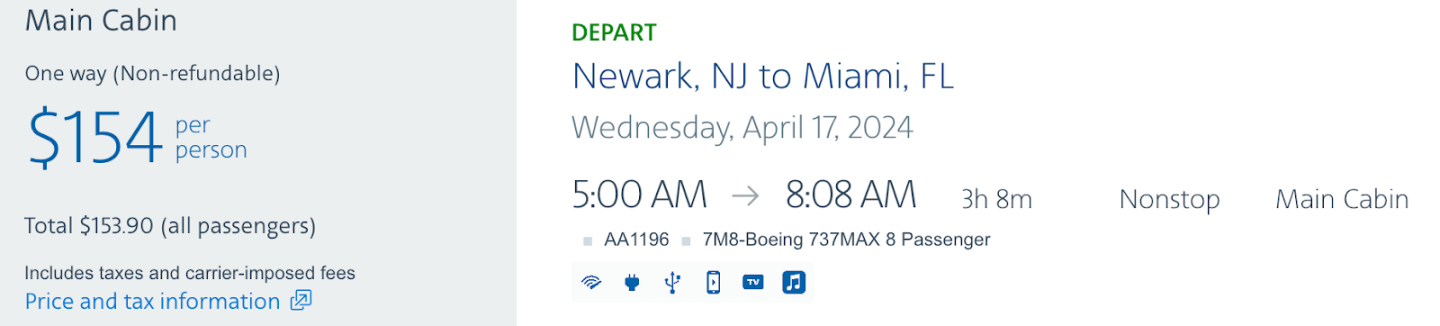
Return flight
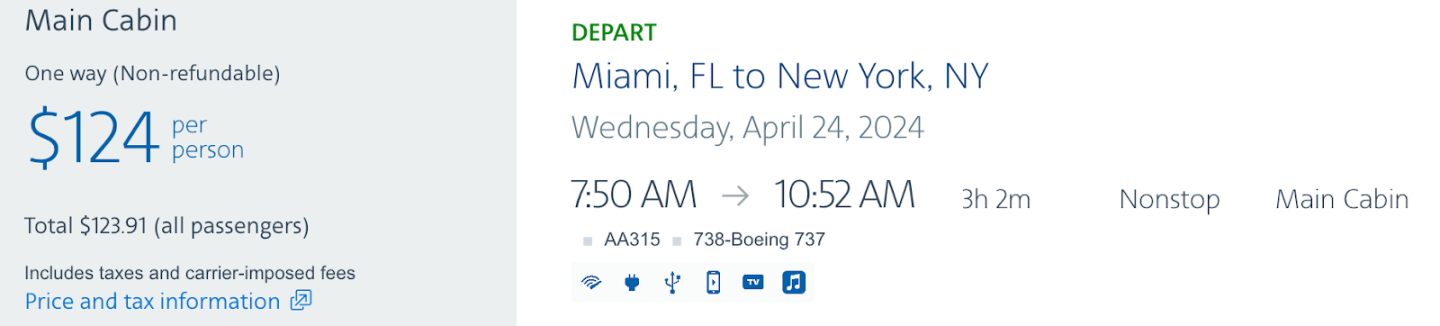
If you were to book these two flights as a round-trip ticket, the price would be exactly the same at $278.
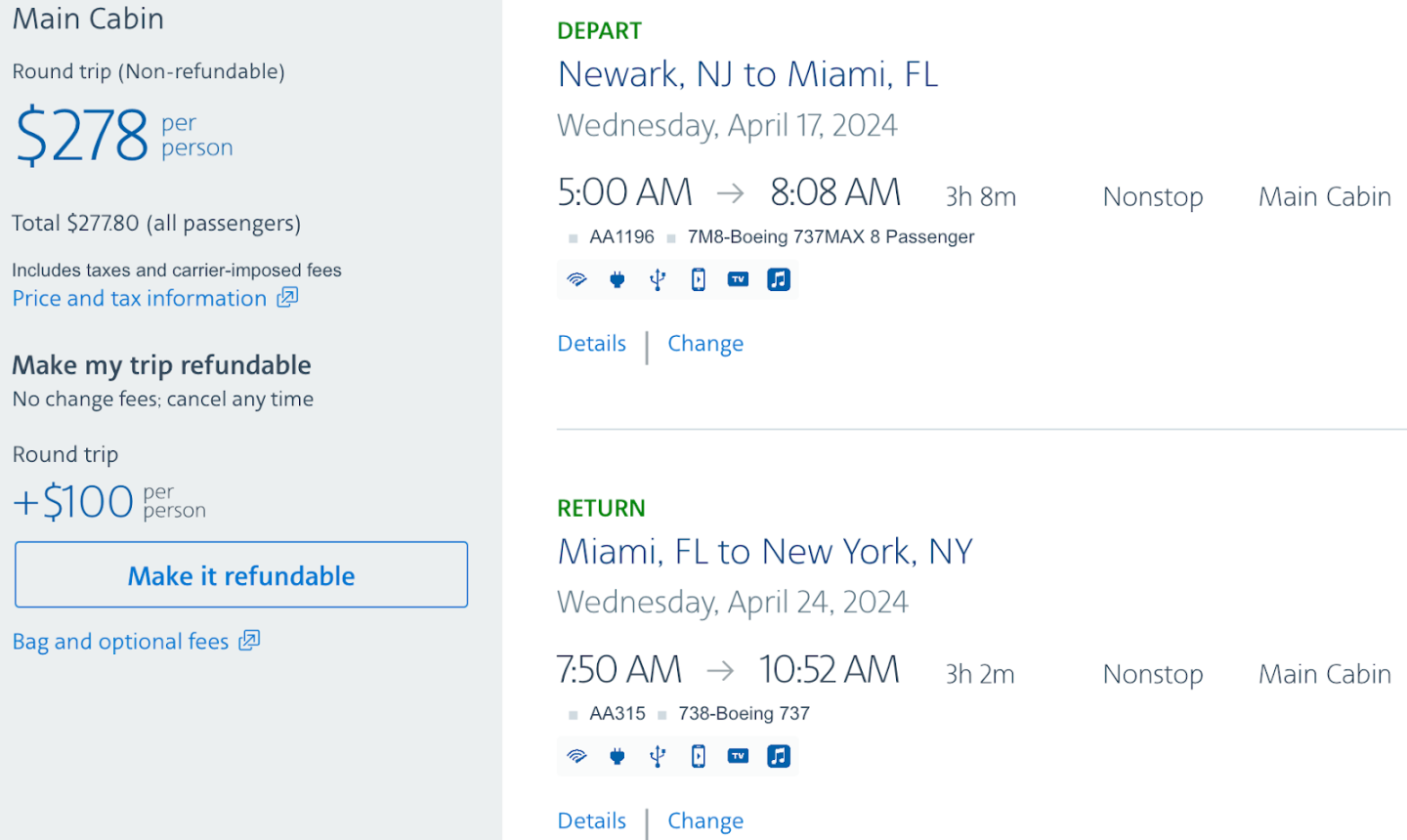
Sample international flight
It's a different ball game with international flights.
Take a look at this flight search from Washington-Dulles to Frankfurt, Germany, on United Airlines . If you were to book the flights as two one-way tickets, it would cost you $3,110.
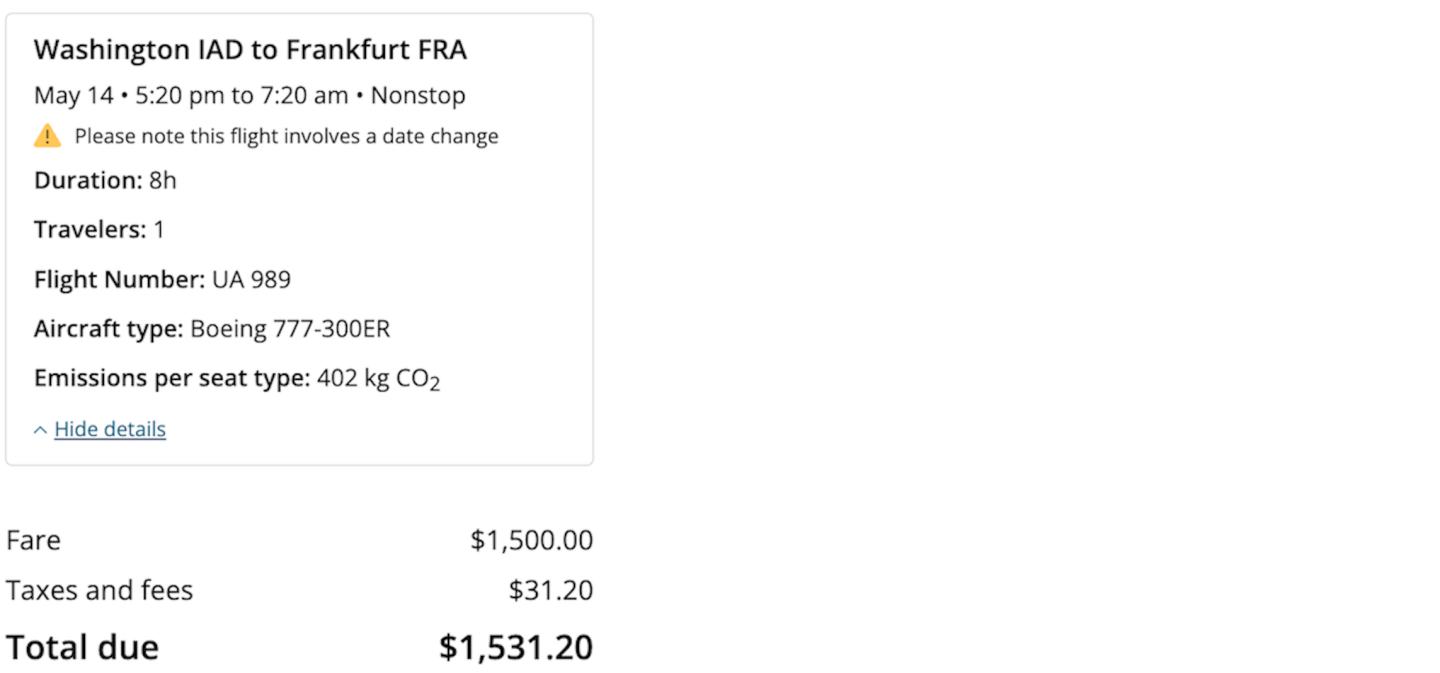
However, if you book these same flights as part of a round-trip ticket, you’d have to pay $1,783, representing a significant savings over booking two one-way flights.
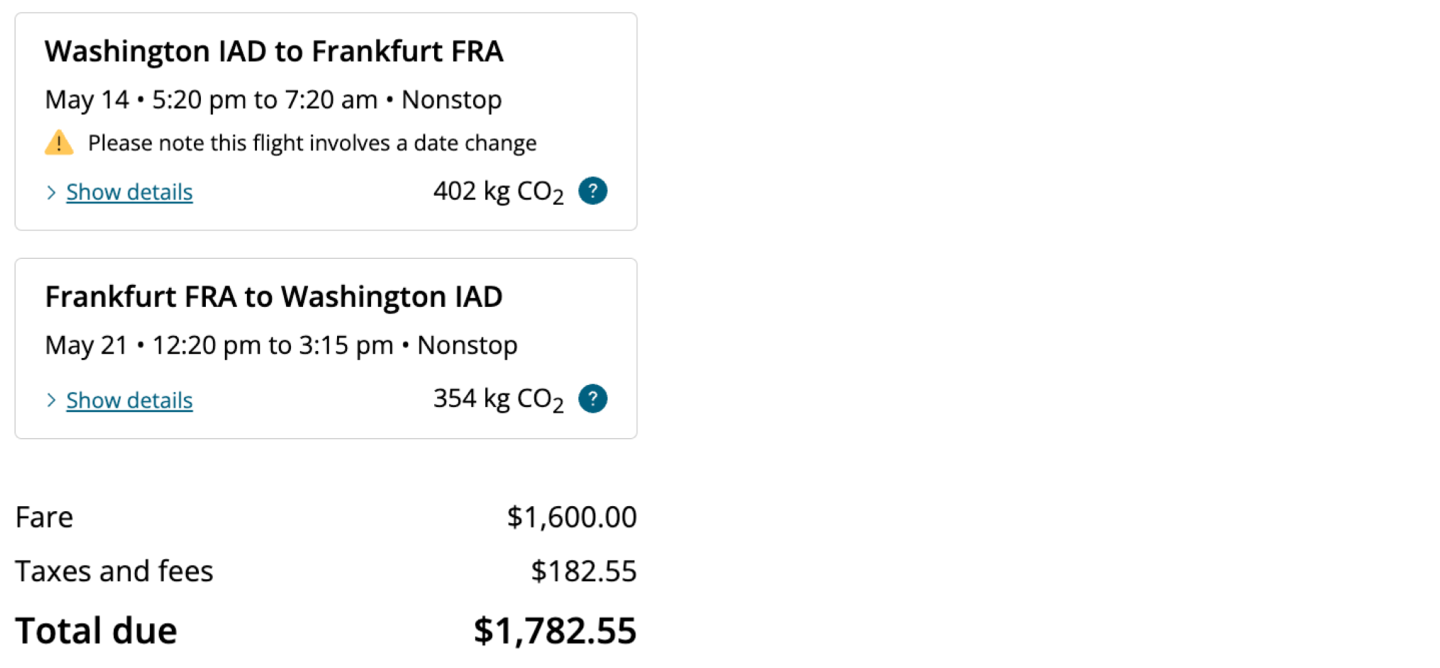
» Learn more: Should I be loyal to a single airline?
If you're planning on using points and miles to book award flights, you have a different set of factors to consider. In the case of award travel, it can make sense to book two one-way flights instead of a round-trip award.
Two one-way awards offer more flexibility
Most U.S. airlines have dropped change fees on both award and paid flights. So, if the price is the same, consider booking two one-way awards instead of a round-trip award to gain extra flexibility. Keep in mind that if you book a round trip, depending on the airline, you may have difficulty getting your miles redeposited if you need to change your award ticket after taking the first leg of the flight.
For example, if you cancel a United award flight midtrip, you'll retain the miles as a future flight credit. While the miles won't be refunded to your account, you'll have the chance to use them in the future.
Watch for higher taxes and fees when booking two one-way awards
When considering whether to book round-trip versus one-way awards, it's important to price out both options — particularly when flying overseas. Some airlines charge higher taxes and fees for awards that originate in certain regions of the world.
Delta SkyMiles is infamous for charging higher fees for award flights that originate in Europe. Also, particularly for international flights, Delta will often price round-trip award flights at less than the cost of two one-way awards.
For example, take a round-trip award from New York to Paris. Delta charges 50,000 SkyMiles plus $85 in taxes and fees for a round-trip main cabin award.
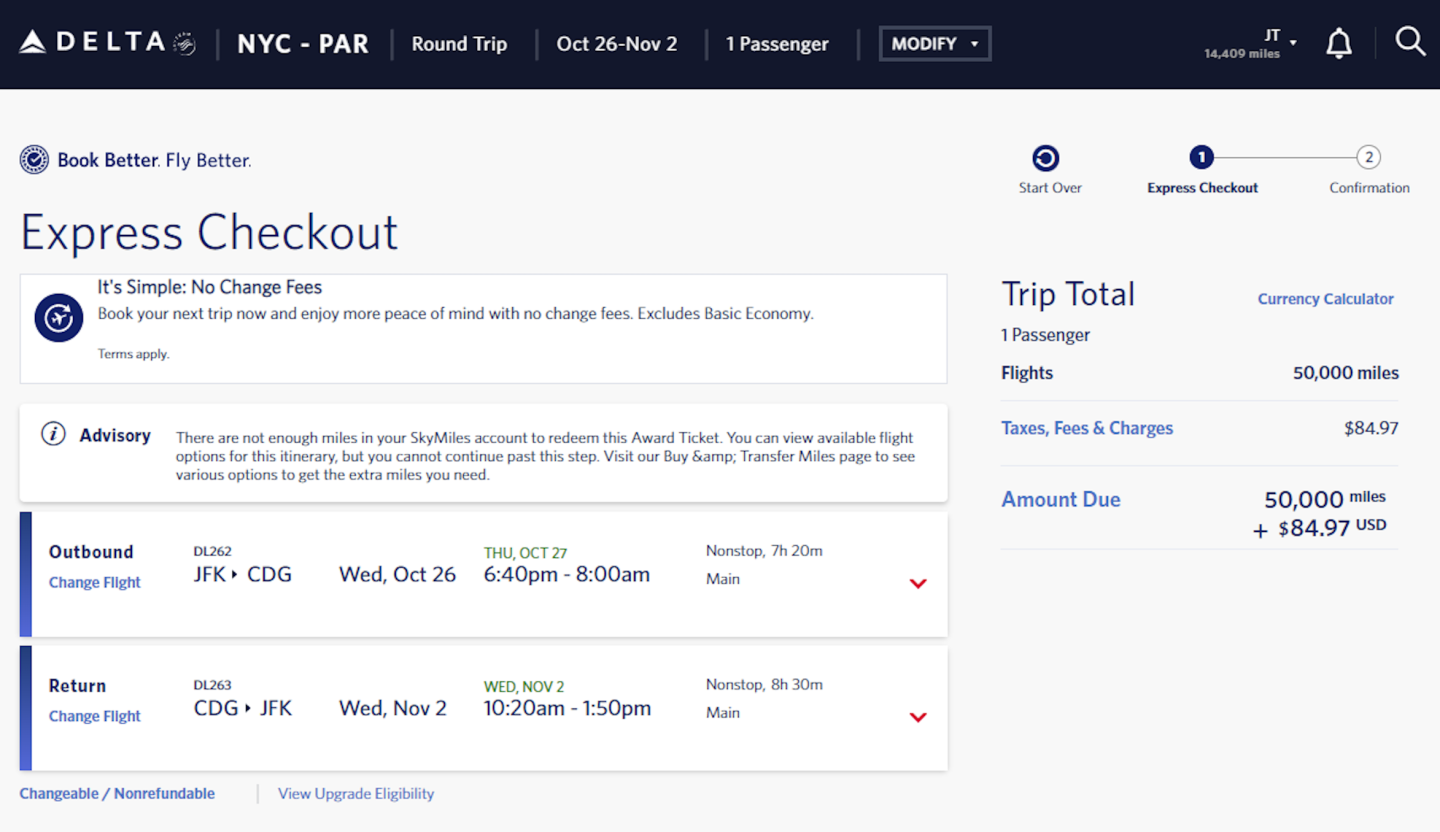
However, if you book the same flights as two one-way awards, you'll pay a total of 74,000 SkyMiles plus roughly $220 in taxes and fees. The outbound award costs 37,000 miles plus $6 in taxes and fees. Then, you'll need to pay another 37,000 SkyMiles plus around $220 to fly home.
In a situation like this, it makes sense to book a round-trip award. You'll save on miles and out-of-pocket costs.
Consider change fees on award flights
Most U.S. airlines have eliminated change fees on both paid flights and award tickets. However, that's not the case with all mileage programs. If you're booking through a mileage program that charges change fees, you may want to book a round-trip award to reduce the fees you could pay if you need to cancel the trip.
» Learn more: Booking flights with points or miles expands refund option
Say you're considering flying to Europe to take a cruise from Budapest to Munich. One might assume that your only options are to:
Book a round-trip flight into one of the cities plus book a one-way intra-Europe flight between them.
Book two one-way flights — one-way into Budapest and one-way back from Munich.
However, there's a third option: booking an "open-jaw" flight . Many airlines will let you book a flight into Budapest and a flight back from Munich on the same ticket. Rather than pricing these flights as two one-way flights, the airline generally prices the flights similar to a round-trip.
» Learn more: The guide to open-jaw flights with Chase points
If you're using airline miles to book these flights, check to see if it's cheaper to book two one-way awards or an open-jaw award. As outlined in the Delta example above, award flights originating in Europe can have much higher taxes and fees. So it might make sense to book the two award segments together as a multicity award.
In the past, airlines charged more for booking two one-way flights rather than a round-trip on the same route. However, this isn't the case anymore on most domestic flights and even some international flights — mostly thanks to competition from low-cost carriers.
In short, airlines charge more for one-way flights on the routes when they can get away with doing so. If only one airline is operating a particular route, it might increase the price of one-way flights to generate more revenue from business travelers and others that are willing to pay more for the nonstop flight.
However, when there's competition from other airlines, the cost of one-way flights often drops to compete with the other airlines serving that route.
Booking a round-trip flight can be cheaper than booking one-way tickets on the same flights. Before booking two one-way flights, check the round-trip price to see if it's cheaper. Even if you're flying out of a different city than you're flying into, booking a so-called "open-jaw" flight could be much cheaper than booking two one-way flights.
Most U.S. airline loyalty programs will charge the same number of miles whether you book round-trip or two one-way flights. Because of this, it can make sense to book two one-way flights instead of a round-trip award to get flexibility in case you need to change or cancel plans.
However, this isn't always the case. Some loyalty programs — such as Delta — charge fewer miles for booking a round-trip award instead of two one-way flights. We recommend pricing out award flights both ways just to be sure.
airline loyalty programs
will charge the same number of miles whether you book round-trip or two one-way flights. Because of this, it can make sense to book two one-way flights instead of a round-trip award to get flexibility in case you need to change or cancel plans.
How to maximize your rewards
You want a travel credit card that prioritizes what’s important to you. Here are some of the best travel credit cards of 2024 :
Flexibility, point transfers and a large bonus: Chase Sapphire Preferred® Card
No annual fee: Wells Fargo Autograph℠ Card
Flat-rate travel rewards: Capital One Venture Rewards Credit Card
Bonus travel rewards and high-end perks: Chase Sapphire Reserve®
Luxury perks: The Platinum Card® from American Express
Business travelers: Ink Business Preferred® Credit Card
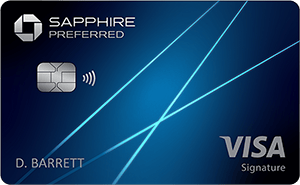
on Chase's website
1x-5x 5x on travel purchased through Chase Travel℠, 3x on dining, select streaming services and online groceries, 2x on all other travel purchases, 1x on all other purchases.
60,000 Earn 60,000 bonus points after you spend $4,000 on purchases in the first 3 months from account opening. That's $750 when you redeem through Chase Travel℠.
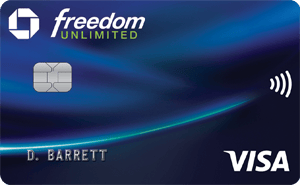
1.5%-5% Enjoy 5% cash back on travel purchased through Chase Travel℠, 3% cash back on drugstore purchases and dining at restaurants, including takeout and eligible delivery service, and unlimited 1.5% cash back on all other purchases.
Up to $300 Earn an additional 1.5% cash back on everything you buy (on up to $20,000 spent in the first year) - worth up to $300 cash back!
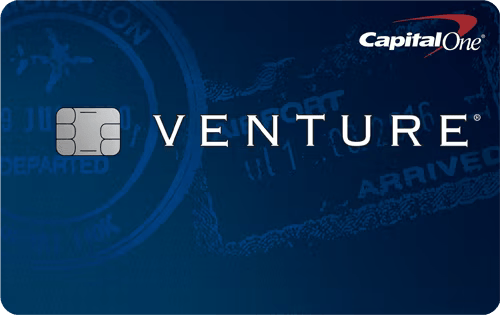
on Capital One's website
2x-5x Earn unlimited 2X miles on every purchase, every day. Earn 5X miles on hotels, vacation rentals and rental cars booked through Capital One Travel, where you'll get Capital One's best prices on thousands of trip options
75,000 Enjoy $250 to use on Capital One Travel in your first cardholder year, plus earn 75,000 bonus miles once you spend $4,000 on purchases within the first 3 months from account opening - that’s equal to $1,000 in travel.
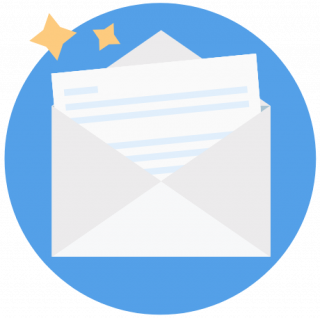

IMAGES
VIDEO
COMMENTS
Round Trip Potential Difference. Since the only points that matter when calculating the potential difference are the initial and final locations, then the round trip potential of any path will equal [math]\displaystyle{ 0 }[/math] since the initial and final locations are the same. Take for example this very simple circuit: Simple Circuit
The round-trip potential difference, which is the round-trip path integral of the electric field, will always add up to zero. A Mathematical Model. Dielectric Constant. Placing an insulator between the plates of a capacitor would decrease the electric field inside the insulator and decrease potential difference across the insulator.
Energy in equals energy out in such a round trip. This makes the amount of work done path independent, and thus the rule you stated (a trivial rule actually that a person by the name of Kirchoff gets credit for stating as a LAW) is true: The sum of the potentials across each of the components must equal the total potential. ... The potential ...
This parallels the idea that round-trip potential difference equals 0, which is incidentally the same as Ampere's Law for Electric Fields. Connectedness. 1. Path independence is a topic that originally stems from multivariable calculus, which has always been one of my favorite subjects in mathematics. I loved studying the connection of so many ...
This means that the largest potential difference that can be measured on this scale is 200 mV. Don't use scales other than V at this time (you could blow a fuse in the meter). ... What is the round trip potential difference from A to B to C to D back to A? Show your calculation, using your mea-
In equation form, the electric potential difference is. The standard metric unit on electric potential difference is the volt, abbreviated V and named in honor of Alessandro Volta. One Volt is equivalent to one Joule per Coulomb. If the electric potential difference between two locations is 1 volt, then one Coulomb of charge will gain 1 joule ...
7.2 Electric Potential and Potential Difference
Khanmigo is now free for all US educators! Plan lessons, develop exit tickets, and so much more with our AI teaching assistant.
It's a sea of charges either way, though. This may support greater currents due to the cross-sectional area but there is little other change. But most of the effect of the dielectric on capacitance happens between the plates. And the round trip path integral of the electric field (the round trip potential difference) must still sum to zero.
See Answer. Question: 4.5 Measuring Voltage Differences in a Two-Bulb Circuit Repeat this round-trip potential difference measurement for a circuit with two batteries placed in the battery holder, two sockets containing round bulbs, and four connecting wires as shown. Record the individual potential differences across each circuit element ...
The loop rule simply states that in any round trip path in a circuit, Electric Potential equals zero. Keep in mind that this applies through ANY round trip path; there can be multiple round trip paths through more complex circuits. This principle deals with the conservation of energy within a circuit.
For example, take a round-trip award from New York to Paris. Delta charges 50,000 SkyMiles plus $85 in taxes and fees for a round-trip main cabin award. However, if you book the same flights as ...
Question. Write a correct energy conservation (round-trip potential difference) equation for this circuit, along a round-trip path starting at the negative end of battery 1 and traveling counterclockwise through the circuit (that is, traveling to the left through the battery, and continuing on around the circuit in the same direction). Solution.
It can sometimes be hard to make sense of the difference between one-way vs. roundtrip flight pricing. In some markets (including most domestic US markets), two one-ways cost the same as a roundtrip. Meanwhile in other markets (including most long haul international markets), booking a roundtrip is much cheaper.
Wi-Fi round-trip time (RTT) ranging has proven successful in indoor localization. Here, it is shown to be useful outdoors as well—and more accurate than smartphone code-based GNSS when used near buildings with Wi-Fi access points (APs). A Bayesian grid with observation and transition models is used to update a probability distribution of the position of the user equipment (UE).
A circuit is constructed from two batteries and two wires, as shown in the diagram below. Each battery has an emf of 1.5 volts.Each wire is 29 cm long, and has a diameter of 0.0007 meters.The wires are made of a metal which has 7e+28 mobile electrons per cubic meter; the electron mobility is 2e-05 (m/s)/(V/m).A steady current runs through the circuit.
A 32.0 nF air-filled parallel plate capacitor is connected in series with a 2.50 kA resistor and a battery of emf 36.0 V. (a) Determine the final charge on the capacitor. с (b) Once the capacitor is fully charged and there is no current in the circuit, a sheet of plastic with a dielectric constant of 2.15 is introduced in the gap between the plates of the capacitor, completely filling the gap.