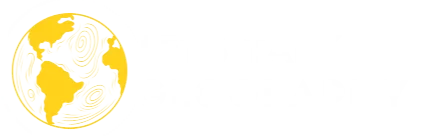

Polar Wandering as Evidence of Continental Drift
Continental drift, once a theory on the fringe of the scientific community, is now a well-established phenomenon. The idea that continents move around on the surface of the Earth has been supported by overwhelming evidence from many different sources. Polar wondering as evidence of continental drift is now a widely known fact. The method of polar wandering uses magnetic data to track how the poles have shifted over time. When overlaid with maps of ancient coastlines, it’s clear that continental drift has occurred many times throughout history.
What is continental drift and how it was first proposed?
When Alfred Wegener first proposed the theory of continental drift in 1912, he did not have enough evidence to convince the scientific community. However, for the next few decades, a growing body of evidence began to support his idea. One such thing is polar wondering as evidence of continental drift.
Polar Wandering is the observed movement of the Earth’s poles over time. This phenomenon can best be explained by continental drift: as the continents move around on the planet’s surface, they drag the poles along with them. Another line of evidence comes from paleomagnetism or the study of ancient magnetic fields. Paleomagnetism has revealed that the Earth’s magnetic field has reversed itself several times throughout its history.
If continental drift were not happening, the poles would be in the same place as they are today and the magnetic field would not have reversed. Together, these lines of evidence provide strong support for continental drift and plate tectonics.
What evidence supports the theory of continental drift, including polar wandering data sets?
Continental drift is a geological theory that suggests the continents on Earth were once one giant landmass, and over time have slowly drifted apart to create the continents we see today. There is evidence that supports this theory, including the occurrence of polar wandering.
Polar wandering occurs when the magnetic North Pole and the South Pole move away from their original positions. This can be traced back through history by studying samples of rock that form in bands, as they contain minerals with different magnetic properties.
When looking at rocks that formed hundreds of millions of years ago, scientists can determine where in the world they were found based on which way they aligned with Earth’s magnetic field at that time. By studying polar wandering data, scientists have been able to confirm continental drift theories. Hence, the scientists approved polar wondering as evidence of continental drift.
Discuss the evidence for polar wandering:
One of the most important pieces of evidence for polar wandering is the fact that the Earth’s magnetic field has reversed itself numerous times throughout the planet’s history. These graphs show how the magnetic pole moves around different continents, and they don’t agree! This is an important finding because it means that all Earth’s landmasses were moving together over time- since there shouldn’t be any difference between them if you look at just one area (like say, Africa).
This evidence is preserved in the rocks, which show a record of the Earth’s magnetic field at the time they were formed. In addition, there are ancient maps that show the continents in different positions than they are today. For example, the Piri Reis map shows Antarctica without ice, proving that it was once located in a different position.
Finally, certain fossils can only be found in specific regions, which suggests that those regions were once located in different locations. All of this evidence points to the fact that the Earth’s poles have wandered over time and establishing polar wondering as evidence of continental drift.
How does continental drift account for geological features on different continents (such as mountains and volcanoes)?
Polar wandering as evidence of continental drift is provided by the observation that the Earth’s poles have not always been in their present locations. Rather, they have “wandered” about over time, as indicated by the changing positions of various magnetic anomalies. The most likely explanation for this phenomenon is that the continents themselves have shifted position over time, carrying the magnetic anomalies with them.
This hypothesis is further supported by the fact that the positions of continental shorelines appear to match up quite well when the continents are reconstructional. For example, the east coast of South America appears to fit quite nicely into the west coast of Africa. Continental drift provides a plausible explanation for the observed geological features on different continents.
What are the potential implications of continental drift on human history/civilization development?
Although the concept of continental drift is now widely accepted by the scientific community, its potential implications on human history are still being explored. The continental drift can have a significant impact on human history and civilization development. For example, when continents move apart, it can create new land masses and alter ocean currents. This can lead to changes in climate , which can impact the development of human societies.
One theory suggests that the breakup of Pangaea played a role in the development of early civilizations. According to this theory, the isolation of landmasses allowed different cultures to develop independently, leading to the formation of distinct societies.
The emergence of new trade routes also played a role in the spread of ideas and technologies between regions. As our understanding of continental drift continues to evolve, we may gain new insights into the origins and development of early civilizations.
Continental drift may also have potential implications for future generations. For example, as sea levels rise , coastal regions will become increasingly vulnerable to flooding and other natural disasters. The continental drift can cause earthquakes and volcanoes.
These natural disasters can destroy infrastructure and disrupt trade routes, potentially leading to the decline of civilizations. Thus, continental drift is a powerful force that has shaped the Earth’s landscape and human history.
Additionally, the shifting of tectonic plates could result in new mountain ranges forming, which could impact global climate patterns. As we continue to learn more about continental drift, we may be able to better prepare for these potential impacts.
How has the study of continental drift evolved, and what challenges remain in this field of research?
The theory of continental drift was first proposed in the early 20th century, and it wasn’t until the 1950s that the theory began to gain acceptance among the scientific community. The main piece of evidence supporting continental drift was the fit of the continents along their edges.
The discovery of plate tectonics in the 1960s provided a possible mechanism for continental drift, and since then the study of continental drift has progressed rapidly. However, there are still many unanswered questions, such as why some plates move faster than others, and what role mantle convection plays in plate tectonics.
Since the early 20th century, the study of continental drift has undergone a dramatic transformation. Initially, the theory was based largely on observations of the physical features of the Earth’s surface. However, as more evidence was gathered, it became clear that there must be an underlying process responsible for the movement of continents.
This led to the development of plate tectonics, which provided a more detailed and accurate explanation for continental drift. Today, plate tectonics is widely accepted as the most likely mechanism for continental drift.
However, there are still some unresolved issues in this field of research. For instance, the researchers lack a mechanism to explain how continents could move. Scientists are still working to determine the exact rate at which continents move.
Additionally, they are also investigating whether or not other planetary bodies, such as Mars, have experienced continental drift. Ultimately, the study of continental drift is an ongoing process, and scientists continue to make discoveries that further our understanding of this phenomenon.
Conclusion:
Continental drift is a real phenomenon that we can see evidence of all around us. It’s amazing to think about how our planet has shifted and changed over time, and it’s thanks to the dedicated efforts of scientists who have pieced together this evidence that we can understand our world in such detail. Have you seen any other compelling evidence for continental drift? Share your thoughts in the comments below!
1. What is polar wandering?
Polar wandering is the shift in the Earth’s poles from one location to another over time. The North and South Poles have not always been located where they are today. For example, during the last ice age, the Earth’s poles were located closer to the equator than they are now.
2. What is Continental Drift?
Continental drift is the scientific theory that explains how the continents have moved over time. The continents are not stationary; they move around on the Earth’s surface. Continental drift occurs when the Earth’s crust (the outermost layer of the Earth) moves. The movement of the continents is very slow, about a few centimeters per year.
3. What is the evidence for polar wandering?
Several lines of evidence suggest that the Earth’s poles have shifted over time. One type of evidence comes from looking at the locations of ancient magnetic stripes on the ocean floor. These stripes are created by lava as it cools and solidifies. The Earth’s magnetic field has reversed many times over the millennia, and these reversals are recorded in the orientation of the magnetic stripes. The stripe pattern shows that the Earth’s poles have moved over time.
4. How does polar wandering help us understand continental drift?
The theory of continental drift proposes that the continents have moved over time. One piece of evidence for this is the fit of the continents like a jigsaw puzzle. For example, the coastlines of Africa and South America fit together perfectly. Another piece of evidence comes from looking at ancient climates. Climates change over time, and certain types of plants and animals can only live in specific climates. If the climate was different in the past, it suggests that the continents have moved to their current locations. Polar wandering is one mechanism that can cause the continents to drift.
5. What is the difference between polar wandering and plate tectonics?
Polar wandering is the shift in the Earth’s poles from one location to another over time. Plate tectonics is the movement and interaction of the Earth’s lithospheric plates. The two phenomena are related, as plate tectonics can cause the continents to drift, which in turn can cause the poles to shift.
6. What are some of the implications of polar wandering?
Polar wandering can have several implications. For example, it can cause climate change, as the shifting of the poles can affect global patterns of atmospheric and oceanic circulation. Additionally, polar wandering can impact navigation, as the Earth’s magnetic field is used to help guide compasses. Finally, polar wandering can cause disruptions to communication systems, as changes in the Earth’s magnetic field can interfere with radio waves.
7. How do we know that polar wandering has happened?
One piece of evidence for polar wandering comes from looking at fossils of animals and plants. Certain types of animals and plants can only live in specific climates. For example, penguins can only live in cold climates near the Earth’s poles. If fossils of penguins are found in areas that were once located near the equator, it suggests that the Earth’s poles have shifted over time.
Sign Up For Daily Newsletter
Be keep up get the latest breaking news delivered straight to your inbox..
Email address:
I loved even more than you could possibly be able to accomplish right here. Despite the fact that the language is stylish and the overall appearance is appealing, there is something odd about the manner that you write that makes me think that you ought to be careful about what you say in the future. In the event that you safeguard this hike, I will most certainly return on multiple occasions.
Thank you for taking the time to share your thoughts on our blog. We’re glad to hear that you find the appearance and style of our content appealing. Your feedback is valuable to us, as it helps us understand our readers’ perspectives and continually improve our blog. We appreciate your advice regarding the manner of our writing. We strive to maintain a balance between being informative and engaging, and it’s important for us to know how our readers perceive our content. Rest assured, we take your comments seriously and will reflect on them as we continue to develop our blog. It’s encouraging to hear that you plan to return to our blog. We look forward to your future visits and hope to continue providing content that meets your expectations and sparks your interest in geography.
Leave a Reply Cancel reply
Your email address will not be published. Required fields are marked *
Save my name, email, and website in this browser for the next time I comment.
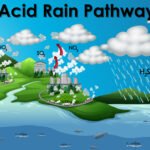
How does Acid Rain affect Climate change?
Discover how acid rain influences climate change in "How does Acid Rain Affect Climate Change?"…
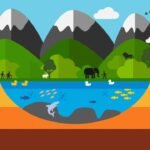
Ecosystem | Definition, Components, and 5 Important Types of Ecosystem
Explore the definition and key components of an ecosystem, along with detailed insights into the…
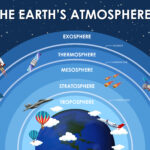
Beyond the Blue: Exploring the 5 Astonishing Layers of the Atmosphere That Protect Our Planet
Unseen Wonders: Discover Earth's 5 Atmospheric Guardians
Sign in to your account
Username or Email Address
Remember Me

- Follow us on Facebook
- Follow us on Twitter
- Follow us on LinkedIn
- Watch us on Youtube
- Audio and video Explore the sights and sounds of the scientific world
- Podcasts Our regular conversations with inspiring figures from the scientific community
- Video Watch our specially filmed videos to get a different slant on the latest science
- Webinars Tune into online presentations that allow expert speakers to explain novel tools and applications
- Latest Explore all the latest news and information on Physics World
- Research updates Keep track of the most exciting research breakthroughs and technology innovations
- News Stay informed about the latest developments that affect scientists in all parts of the world
- Features Take a deeper look at the emerging trends and key issues within the global scientific community
- Opinion and reviews Find out whether you agree with our expert commentators
- Interviews Discover the views of leading figures in the scientific community
- Analysis Discover the stories behind the headlines
- Blog Enjoy a more personal take on the key events in and around science
- Physics World Live
- Impact Explore the value of scientific research for industry, the economy and society
- Events Plan the meetings and conferences you want to attend with our comprehensive events calendar
- Innovation showcases A round-up of the latest innovation from our corporate partners
- Collections Explore special collections that bring together our best content on trending topics
- Artificial intelligence Explore the ways in which today’s world relies on AI, and ponder how this technology might shape the world of tomorrow
- #BlackInPhysics Celebrating Black physicists and revealing a more complete picture of what a physicist looks like
- Nanotechnology in action The challenges and opportunities of turning advances in nanotechnology into commercial products
- The Nobel Prize for Physics Explore the work of recent Nobel laureates, find out what happens behind the scenes, and discover some who were overlooked for the prize
- Revolutions in computing Find out how scientists are exploiting digital technologies to understand online behaviour and drive research progress
- The science and business of space Explore the latest trends and opportunities associated with designing, building, launching and exploiting space-based technologies
- Supercool physics Experiments that probe the exotic behaviour of matter at ultralow temperatures depend on the latest cryogenics technology
- Women in physics Celebrating women in physics and their contributions to the field
- IOP Publishing
- Enter e-mail address
- Show Enter password
- Remember me Forgot your password?
- Access more than 20 years of online content
- Manage which e-mail newsletters you want to receive
- Read about the big breakthroughs and innovations across 13 scientific topics
- Explore the key issues and trends within the global scientific community
- Choose which e-mail newsletters you want to receive
Reset your password
Please enter the e-mail address you used to register to reset your password
Note: The verification e-mail to change your password should arrive immediately. However, in some cases it takes longer. Don't forget to check your spam folder.
If you haven't received the e-mail in 24 hours, please contact [email protected]
Registration complete
Thank you for registering with Physics World If you'd like to change your details at any time, please visit My account
- Planetary science
- Research update
How Earth’s wandering poles return home
A number of times over the past one billion years, the Earth’s surface has “wandered” relative to its rotational axis – before returning to its original position. Now, a team of geophysicists from the US and Canada says it has developed a theory that explains this curious phenomenon of “oscillatory true polar wander”. Understanding the mechanics behind polar wander is crucial, as a shift could tip the Earth over by as far as 50° over a period of 10–100 million years and this would cause profound global environmental and geological changes.
True polar wander (TPW) can be defined as the relative movement between the mantle (and so the surface of the Earth) and the Earth’s spin axis or its rotational axis. Incredibly, researchers believe that over the past one billion years, the Earth’s surface has “tipped over” and then returned to its original location six times along the same axis – this is the process of “oscillatory true polar wander”. Scientists have worked this out by studying magnetism in rocks – a discipline known as “paleomagnetism”. If a rock cools in a magnetic field, it records the magnetic properties of the field and these can be decoded in the lab millions of years later. So, by measuring changes in the orientation of the Earth’s magnetic field that are stored in ancient rocks, scientists can “see” the effects of the oscillatory TPW.
Extreme shifts
“Someone sitting on the Earth would have seen the pole shift up to 50° and then turn around and return close to its original location, all in tens of millions of years,” explains geophysicist Jerry Mitrovica of the Earth and Planetary Science Department at Harvard University. “But an observer floating in space would actually see the rotational axis stay relatively vertical and the Earth’s surface tip over and then back.” Unsurprisingly, these rather extreme and dramatic shifts can be linked to global changes in all large-scale Earth systems such as the carbon cycle, climate and even evolution. “After all, if it happened today, a shift of 50° one way might put Boston [Massachusetts] near the north pole, while a shift in the opposite direction would bring Boston near the equator,” says Mitrovica.
But this in itself is not news – earth scientists have known for a while that TPW does occur and they even know why. They believe that the initial shift of the pole – or the Earth tipping over – is caused by large-scale flows in the Earth’s interior known as “mantle convection”, involving thermal convection currents that carry heat from the Earth’s core to the surface. This is the same process that drives continental drift and plate tectonics. So, mantle convection disturbs the rotational equilibrium of the Earth and the result is a shift in the relative orientation of the Earth’s solid surface and its rotational axis.
There and back again
What has eluded researchers is a theory that clearly explains how and why the pole returns to its original location, or the “oscillatory true polar wander”. In the new work, graduate student Jessica Creveling, also of the Earth and Planetary Science Department at Harvard, along with Mitrovica and colleagues, provides an explanation. The researchers, using computer simulations and modelling, say that a combination of two mechanisms brings the “wandering” pole back to its original location.
The first mechanism relates to the Earth’s equatorial bulge. The Earth is not a perfect sphere – rather it is an oblate spheroid, as it is flattened at the poles and bulges at the equator. So there is a difference in the radius of the Earth as measured from the centre to the equator compared with the poles – it is approximately 20 km greater at the equator. This band of excess mass forms because the Earth is rotating, which causes the equator to bulge outwards. “But the Earth’s bulge is generally a bit larger than it should be…which is true even today. And this extra bulge, or fatness, acts to stabilize the Earth’s rotation,” explains Mitrovica. He likens this to the heavy weight that is placed at the bottom of a plastic punching-bag toy, which acts to bring the bag back to being vertical if it is punched sideways. In a similar manner, if the Earth, with its bulging equator, tips over, it prefers to right itself again. “So, this girdle of excess mass actually has a very stabilizing effect, acting as a self-righting mechanism for the Earth’s rotation,” he says.
The second mechanism relates to the strength of the tectonic plates. If the Earth’s surface tips over relative to the rotational axis, the 12 larger tectonic plates all get deformed to a small extent, like elastic bands. In a similar way to a stretched elastic band, the plates want to go back to their original size, and these stabilizing elastic stresses also play a role in the oscillatory return of the pole. A clue that this might be the case is the fact that past polar-oscillation events seem to have happened when the Earth’s continents were gathered together into one “supercontinent”, a process that has repeated a number of times in Earth’s history. The last supercontinent, known as Pangea, was formed 200 million years ago.
Efficiency of combined effects
Mitrovica points out that while Creveling was running her simulation, neither single mechanism could cause the pole to return – it was only a combination of both effects that did it. “What also really surprised me was the efficiency of the effects to pull and push the poles during a period of about 10 million years,” says Mitrovica. “This paper made a believer out of me and I was a sceptic.” He explains that other researchers might remain sceptical about the theory and that only more evidence gathered based on paleomagnetic field studies will provide the necessary evidence. The team also hopes to better determine how common or rare these events are. “Every rock cooling at the time of a tilt will show the evidence of it and we need to find that,” says Mitrovica.
The team is also keen to determine just how drastic the effects of a shift are. It is believed that a shift would cause a significant change in the climate of every place on Earth, as well as changes in the sea level and the carbon cycle. Mitrovica believes that the consequences of these large-scale events would have left their own mark on the Earth’s systems and they too should be studied in the future.
The research was published in Nature .
Want to read more?
Note: The verification e-mail to complete your account registration should arrive immediately. However, in some cases it takes longer. Don't forget to check your spam folder.
If you haven't received the e-mail in 24 hours, please contact [email protected] .
- E-mail Address

Physics World Jobs
Take the next step in your career and find your perfect job
- Nanomaterials
Nanocrystals produce hydrogen using sunlight
Vibrating molecule drives a motor, discover more from physics world.
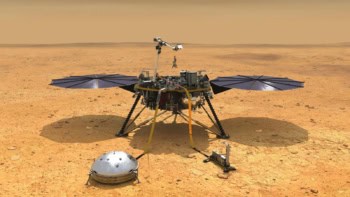
Liquid water could abound in Martian crust, seismic study suggests

- Astronomy and space
NASA cancels delay-hit $450m VIPER lunar prospector
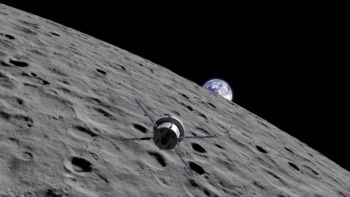
- Telescopes and space missions
Atomic clocks on the Moon could create ‘lunar positioning system’
Related jobs, assistant professor/postdoctoral research fellow, senior vacuum engineer, postdoctoral associate -observational extragalactic astrophysics and cosmology, related events.
- Particle and nuclear | Symposium Fifth International Symposium on the Casimir Effect 15—21 September 2024 | Piran, Slovenia
- Astronomy and space | Conference Seventh International Conference on the Nature and Ontology of Spacetime 16—19 September 2024 | Albena, Bulgaria
- Astronomy and space | Exhibition ANE Global Meet and Expo on Astronomy, Astrophysics and Cosmology 7—9 April 2025 | London, UK
How Earth's Outer Layers Wander Back and Forth
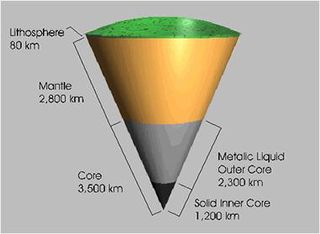
The entire outermost part of Earth is able to wander over the rest of the planet, and now researchers say in a new study detailed in the Nov. 8 issue of the journal Nature that they can explain how it can mysteriously return back the way it was.
The planet's solid exterior — its crust and most of its mantle layer — at times drifts over its core. To envision this, imagine that a peach's flesh somehow became detached from the fruit's pit and was free to move about over it.
These shifts can prove rather extreme. A person sitting on the Earth could have seen the pole apparently wander up to 50 degrees and then return close to its original location, all in tens of millions of years.
"If it happened today, a shift of 50 degrees one way might put Boston near the north pole, while a shift in the opposite direction would bring Boston near the equator," said study researcherJessica Creveling, a geologist and geochemist now at the California Institute of Technology. "Not surprisingly, these dramatic shifts have been linked to major changes in nearly all aspects of the Earth system, including the carbon cycle, climate, evolution." [ Earth Quiz: Mysteries of the Blue Marble ]
Wandering poles
This movement of Earth's outermost layers is known as " true polar wander ." It differs from the motions of the plates making up Earth's crust, known as plate tectonics .
Scientists think they understand the processes behind the original shift in the poles — that is, what causes the tip-over.
Sign up for the Live Science daily newsletter now
Get the world’s most fascinating discoveries delivered straight to your inbox.
"This shift is due to large-scale flow in the Earth's rocky interior known as mantle convection, the same process that drives continental drift and plate tectonics," Creveling said. These include "superswells" beneath southern Africa and the Pacific Ocean, which cause the Earth underneath them to lift.
However, the reasons the poles returned to their original locations remained a mystery.
"This is the most puzzling aspect of all," said study researcher Jerry Mitrovica, a geophysicist at Harvard University. "Why would the Earth return to its original orientation?"
Now the researchers suspect they might have two answers to this puzzle that have to do with the shape of the Earth and the elasticity of its tectonic plates.
Earth's bulge
Computer simulations run for the study first looked at the planet's equatorial bulge — that is, the way Earth swells around the middle.
"This increased girth is due to the Earth's rotation, which causes the equator to bulge outwards," Creveling said.
The Earth's bulge is a bit larger than one might expect just from its rotation due to the flow of rock in Earth's mantle layer.
"This extra bulge or fatness acts to stabilize the Earth's rotation just like the heavy weight at the bottom of a plastic punching bag toy will act to bring the bag back to being vertical if it is punched sideways," Creveling said. "The excess bulge of the Earth acts as a self-righting mechanism for the Earth's rotation."
Elastic tectonic plates
The computer simulations also looked at the elasticity (a measure of how a material deforms under stress) of Earth's tectonic plates .
"It turns out that if the pole moves on the Earth's surface, the plates all get deformed a little bit, just like small elastic bands," Creveling said. "And just like elastic bands that are stretched, the plates will want to go back to their original size. This elastic strength may have also played a role in the oscillatory return of the pole in the dramatic true polar wander events."
One piece of evidence suggesting this elasticity plays a role in the return trip of the poles "is the fact that these tip-over-and-back events seemed to happen when the Earth's continents were gathered together into one 'supercontinent,' a process that we know has repeated a number of times in Earth history," Creveling said. (The last supercontinent, which existed 200 million years ago, was called Pangaea).
Mitrovica noted that the current shape of the Earth and the fact that it now has lots of tectonic plates and spread-out continents means that conditions are not favorable for a tip-over event.
"It won't happen again anytime soon," Mitrovica told OurAmazingPlanet.
Future research can investigate how rare or how common these polar wander events were.
"Once this is better established, it will be important to study the way in which the Earth system was impacted," Mitrovica said.
This story was provided by OurAmazingPlanet , a sister site to LiveScience. Follow OurAmazingPlanet for the latest in Earth science and exploration news on Twitter @OAPlanet . We're also on Facebook & Google+ .
Yarlung Tsangpo: The deepest canyon on land hides a tree taller than the Statue of Liberty
Scientists drill longest-ever piece of Earth's mantle from underwater mountain near 'Lost City'
Rare Roman-era silver ingots depicting Constantine the Great seized from alleged black-market sale
Most Popular
- 2 Mpox outbreak in Africa could swell into a pandemic — here's how we stop it
- 3 Perseid meteor shower rains 'shooting stars' over Stonehenge in glorious astrophotography image
- 4 Stonehenge's 13,200-pound Altar Stone originated in northeastern Scotland, more than 450 miles away
- 5 Chinese rocket breaks apart after megaconstellation launch, creating cloud of space junk
Thank you for visiting nature.com. You are using a browser version with limited support for CSS. To obtain the best experience, we recommend you use a more up to date browser (or turn off compatibility mode in Internet Explorer). In the meantime, to ensure continued support, we are displaying the site without styles and JavaScript.
- View all journals
- My Account Login
- Explore content
- About the journal
- Publish with us
- Sign up for alerts
- Open access
- Published: 26 December 2022
Ordovician–Silurian true polar wander as a mechanism for severe glaciation and mass extinction
- Xianqing Jing 1 ,
- Zhenyu Yang ORCID: orcid.org/0000-0003-1388-2519 1 ,
- Ross N. Mitchell ORCID: orcid.org/0000-0002-5349-7909 2 ,
- Yabo Tong 3 ,
- Min Zhu ORCID: orcid.org/0000-0002-4786-0898 4 &
- Bo Wan ORCID: orcid.org/0000-0002-5896-9485 2
Nature Communications volume 13 , Article number: 7941 ( 2022 ) Cite this article
9816 Accesses
11 Citations
25 Altmetric
Metrics details
- Geodynamics
- Palaeomagnetism
- Palaeontology
The Ordovician–Silurian transition experienced severe, but enigmatic, glaciation, as well as a paradoxical combination of mass extinction and species origination. Here we report a large and fast true polar wander (TPW) event that occurred 450–440 million years ago based on palaeomagnetic data from South China and compiled reliable palaeopoles from all major continents. Collectively, a ~50˚ wholesale rotation with maximum continental speeds of ~55 cm yr −1 is demonstrated. Multiple isolated continents moving rapidly, synchronously, and unidirectionally is less consistent with and plausible for relative plate motions than TPW. Palaeogeographic reconstructions constrained by TPW controlling for palaeolongitude explain the timing and migration of glacial centers across Gondwana, as well as the protracted end-Ordovician mass extinction. The global quadrature pattern of latitude change during TPW further explains why the extinction was accompanied by elevated levels of origination as some continents migrated into or remained in the amenable tropics.
Similar content being viewed by others

Completing the loop of the Late Jurassic–Early Cretaceous true polar wander event

Quantitative comparison of geological data and model simulations constrains early Cambrian geography and climate
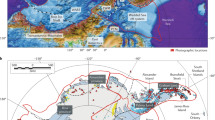
The geological history and evolution of West Antarctica
Introduction.
The Earth system underwent critical changes during the Ordovician–Silurian (O–S) transition 460–435 million years (Ma) ago. The end-Ordovician mass extinction, which can be regarded as the second most lethal of the “Big Five” mass extinctions, replaced much of the Cambrian marine fauna with later Paleozoic fauna 1 . Accompanying the O–S mass extinction was the first of three occurrences of significant glaciation during the Phanerozoic Eon (ca. 541 Ma to present), of which only ~25% represented glacial intervals 2 . The O–S glacial interval is unusual both because it was short-lived and occurred at a higher atmospheric partial pressure of CO 2 , perhaps 8–16 times higher than today 3 . There are multiple hypothesized causes for the Ordovician extinction, including intense volcanic eruptions and/or large igneous provinces 4 , 5 , 6 , 7 , oceanic anoxia 4 , 6 , special paleogeography 8 , large and short-lived glaciation 8 , 9 , 10 , and even the evolution of land plants 9 , 10 . The diversity of proposed mechanisms thus reflects the myriad changes in the atmosphere, biosphere, hydrosphere, lithosphere, as well as in the mantle at that time.
Among the candidate mechanisms behind the widespread O–S global change, intense volcanism and paleogeography are generally regarded as the basic causes for the other changes 4 , 6 , 7 , 8 . However, there is still debate over how exactly volcanism impacted the environment 4 , with some arguing that it resulted in global warming, while others claiming it caused glaciation. The volcanism theory has also been used to explain the traditional two-pulse extinction model 5 , but recently reported high-resolution biodiversity curves 1 , 7 , 11 suggest instead a protracted extinction rather than the simple traditional two-pulse model. Therefore, the mechanisms once fit to a two-pulse extinction model may no longer be applicable, or at least require modification.
Paleogeography is another critical boundary condition for understanding such marked transitions in Earth’s surface environment, but the prevalent palaeogeographic models used 5 , 6 , 8 , 12 are imprecise, lacking palaeolongitude control and high temporal resolution. Constraining palaeolongitude is particularly important when continents are dispersed as they were in the early Paleozoic during the transition between supercontinents Rodinia and Pangaea. Temporal resolution is critical when continents are moving fast and multiple kinematic models suggest some of the highest continental motions of the Phanerozoic Eon occurred during this time 13 . It is therefore difficult to evaluate the exact impact palaeogeographic changes may have had on the end-Ordovician environmental changes. For example, employing prevalent palaeogeographic models, biogeochemical models 14 fail to both recreate the environmental changes during the critical Hirnantian stage as well as to explain the migration of the glacial centers 15 , 16 .
Similar extreme transitions in Earth’s surface conditions occurred during the preceding Ediacaran–Cambrian periods, and this interval has been proposed to have experienced large-scale (60–90˚) true polar wander 17 , 18 , 19 , 20 , 21 , 22 , 23 . True polar wander (TPW) is the movement of the entire solid Earth (mantle and crust) relative to Earth’s spin axis in order to stabilize Earth rotation. It is different from the plate motion of plate tectonic theory, which proposes that tectonic plates, including continents or not, move over the asthenosphere relative to the underlying convecting mantle. Tectonic plates move in different directions and with different velocities (even in the case symmetric seafloor spreading, the directions of motion are opposite of each other). In contrast, TPW can induce wholesale polar motion of the plates unidirectionally and synchronously, thus changing paleogeography rapidly and globally. Therefore, TPW potentially impacts much of Earth system evolution including changing ocean currents, air circulation, relative sea level, and depocenters of the carbon cycle 18 , 21 , 24 , 25 , 26 .
Van der Voo 27 first proposed a round-trip TPW oscillation (two sequential back-and-forth TPW events) during the Late Ordovician to Late Devonian based on sparse and roughly-dated palaeomagnetic poles from three continents exhibiting similar large and rapid segments of apparent polar wander (APW) 27 . The proposed ~40˚ amplitude of the putative O–S TPW event (the first event of the pair in the oscillation), if shown to be valid, would represent the largest TPW event in the past 500 million years 28 . Although Piper et al. 29 revisited this TPW interval, they only studied the late Silurian–Early Devonian part. No follow-up research has reexamined the Ordovician–Silurian TPW event, which, as proposed, is too crude to assess its validity nor its potential impact on the environmental and ecological changes occurring at that time. Many new palaeomagnetic results during this purported interval of large-scale TPW have been reported since 30 , 31 , 32 , 33 , 34 , justifying a reexamination. Furthermore, although putative O–S TPW was proposed 27 prior to most of the numerous hypotheses attempting to explain the variegated aspects of global change during this transition, O–S TPW has never before been taken into account for its potential environmental effects. Even for those models involving palaeogeography as a critical aspect for the changing surface conditions during this dramatic environmental transition, TPW has been neglected.
In this work, we present palaeomagnetic data from South China as well as compile data globally from 6 continents to provide a rigorous and high-resolution palaeogeographic reconstruction of the O–S transition. Our results demonstrate the occurrence of a large and rapid TPW rotation synchronous with the environmental changes across the O–S boundary. The heretofore enigmatic features of global change during this time interval can collectively be reconciled by this refined TPW-based palaeogeographic model, explaining both glacial and extinction dynamics.
New palaeomagnetic data from South China
Previous palaeomagnetic data from South China tentatively suggest there may have been a rapid continental movement during the Late Ordovician to early Silurian 30 , 32 , 35 . However, data from the Silurian have been calculated as a mean pole for the whole Period (443.8–419 Ma) 32 , 35 (Supplementary Fig. 1 ), which precludes detailed evaluation of maximum rates of continental motion during the O–S transition. Due to its importance for palaeogeographic comparison before and after the O–S boundary, the upper Telychian strata of the Huixingshao Formation (ca. 436–435 Ma) in Xiushan county, Chongqing, South China (Supplementary Figs. 1 and 2 ) were selected for detailed palaeomagnetic study. Standard palaeomagnetic methods were employed and are detailed in the Methods. Stepwise thermal demagnetization revealed a stable component with high unblocking temperature suggestive of a remanence carried by hematite, which is also supported by rock magnetic experiments (Fig. 1 and Supplementary Figs. 3 , 4 , and 6 ). Detailed description of the palaeomagnetic results is provided in the Supplementary information. The magnetostratigraphic record reveals at least four coherent polarity zones (Fig. 2 ) strongly suggesting that the high-temperature component from section Yongdong (SY) is primary and can be used for palaeogeographic reconstructions. However, the K- value of dispersion of the virtual geomagnetic poles (VGPs) of these six sites is 90.3 (Supplementary Table 1 ), exceeding 70, which suggests that these data may not average out the palaeosecular variation (PSV) 36 . To overcome this issue, we sought to combine our new data with the most reliable coeval previous data.
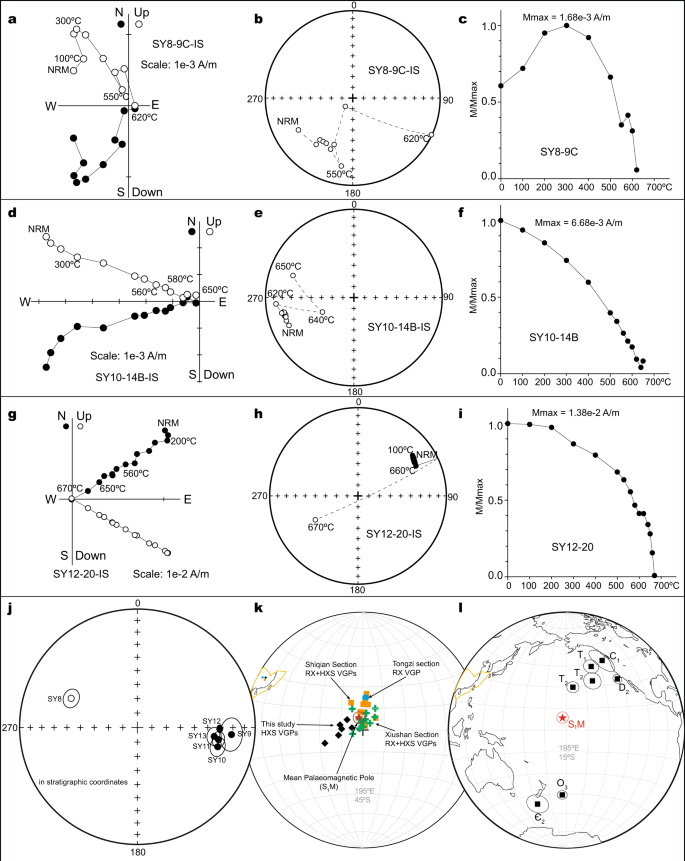
Zijderveld plots ( a , d , g ), equal area projections ( b , e , h ) and normalized stepwise thermal decay curves ( c , f , i ) of the thermal demagnetization of representative samples from the section at Yongdong (SY) in geographic coordinates. In the Zijderveld plots, black and white dots represent horizontal and vertical projections, respectively, while in the equal area projections, they represent directions plotted in the lower and upper hemispheres respectively. j Equal area stereographic projection of site mean directions of the high-temperature components of the Huixingshao (HXS) Fm from this study in stratigraphic coordinates. k Virtual geomagnetic poles (VGPs) of HXS Fm from the SY section from this study compared with VGPs from the HXS Fm and the Rongxi (RX) Fm from Opdyke et al. 35 and Huang et al. 32 . Resulting combined early Silurian pole (S 1 M) using all data from the RX and HXS Formations from this study and previous work is shown as red star with associated cone of 95% confidence. l The new recalculated early Silurian pole (S 1 M) is distinct from existing poles of South China 71 . All plots were generated with PaleoMac 72 .
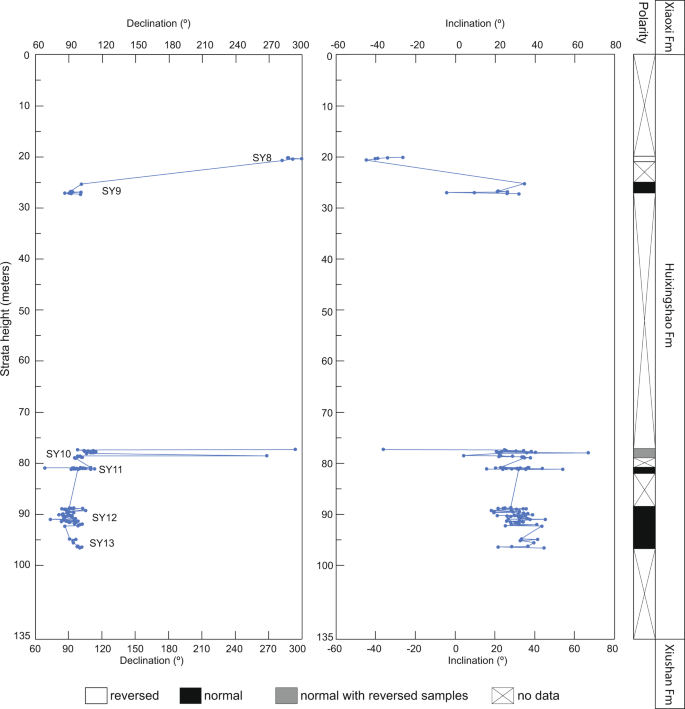
Sampled section at Yongdong (SY). Directions with declinations >240° were interpreted as reversed polarity, and otherwise, as normal polarity.
We reassign the ages of existing Silurian palaeomagnetic results 32 , 35 according to a recently updated stratigraphic timescale 37 , 38 , 39 (Supplementary Fig. 1 ). A notable revision in these age reassignments is that the Rongxi Formation previously regarded as ca. 420 Ma in age is in fact early Telychian (ca. 438.5–437 Ma) (Supplementary Fig. 1 ). Again, data from these previous studies 32 , 35 seem not to average out PSV 36 (Supplementary Table 1 ; detailed analysis in Supplementary information). Nonetheless, after combining all data from the Rongxi and Huixingshao Formations (total 28 sites), a K -value of 48.4 is achieved, which is below 70 and suggests sufficient averaging of PSV. Furthermore, these data also pass a fold test 40 at 99% confidence (k in geographic coordinates is 7.64, in stratigraphic coordinates is 31.17). This new early Silurian pole (S 1 M) calculated by averaging the VGPs from the Rongxi and Huixingshao Formations plots far from all younger poles and earns a reliability index of 6 of 7 (ref. 36 , Supplementary information). Intriguingly, the new early Silurian pole (S 1 M) plots far from (≥50°) away a high-quality Late Ordovician (late Sandbian–middle Katian; 454–448 Ma, or ca. 451 Ma) pole of South China 30 (Figs. 1 l, 3a ).
Given only ~10 Ma between these two ages, the 54.4° ± 6.4° arc distance between these two poles indicates a rapid APW rate of 5.4 ± 0.6 Ma −1 for South China. During this time interval, South China experienced a region tectonic movement, however it was restricted to only its southeastern part (Cathaysia terrane) 41 . Our early Silurian data and the Late Ordovician data are from northwestern South China (upper Yangtze terrane), which was largely unaffected by this tectonism. In addition, the regional tectonism should have only induced large differences in the declination of these data (due to potential vertical-axis rotation), but cannot explain the large inclination difference that is observed corresponding to a ~28.5° change in palaeolatitude. Non-uniformitarian magnetic fields (e.g., quadrupolar or octupolar) may also result in apparent changes in latitude 33 . However, in order to explain the reduced inclination of the Late Ordovician data (35°) to our Silurian data (18°), one would have to claim a same-sign octupole that was stronger than 20%, which is more extreme than any previous claims in the Phanerozoic 42 , and an opposite-sign octupole would increase, not decrease, inclination. Furthermore, both non-dipole cases would only affect inclination and therefore cannot explain the even larger anomaly in terms of the ~59° declination change. Lastly, an oscillation between polar and equatorial dipoles (if possible on Earth) could affect declination 43 , but would predict a ~90˚ change that is not observed. Therefore, we argue that this large and rapid motion of South China corroborates from an additional continent the proposed O–S TPW event 27 , albeit with an even larger amplitude than once thought. Nevertheless, any reproducibility test of TPW should aim to be global in scope, so we must consider the palaeomagnetic records of the other major continents.
Late Ordovician-early Silurian true polar wander
Strikingly, in addition to the large-scale 54° ± 6° APW of South China, the Late Ordovician–early Silurian palaeopoles from Tarim, Siberia, Baltica, and Gondwana also all demonstrate large arc distances of APW: 54° ± 9°, 47° ± 17°, 55° ± 14°, and 58° ± 21°, respectively (Fig. 3a , Supplementary Table 2 ), with associated APW rates of 5.4° ± 0.9°, 4.7° ± 1.7°, 5.5° ± 1.4°, and 5.8° ± 2.1° Ma −1 , respectively. Data from Baltica and Gondwana represent recent synthetic APW paths, which consider the age error and the quality of the data 34 . For comparison, we also calculate the arc distances for Baltica and Gondwana from 450–430 Ma using the synthetic APW paths of Torsvik et al. 44 (Supplementary Table 3 ), which are 51.2° ± 8.2° and 24.5° ± 18°, respectively. While the results for Baltica agree with both methods, the large difference of the two synthetic APW paths for Gondwana reflect either the larger 20 Ma age bins of Torsvik et al. 44 oversmoothing the data and/or the lack of poles during this time interval which is non-ideal for synthetic methods. Nonetheless, at least four continents demonstrate similar large amplitude and synchronous polar motion. As discussed, regional tectonics and non-uniformitarian geomagnetic fields cannot explain this systematic global APW anomaly. Plate motion, driven by slab subduction and mantle convection, also cannot explain these synchronous and similar large amplitude movements of multiple isolated continents either, as it requires relative motion between different plates with different velocities (speeds and/or directions).
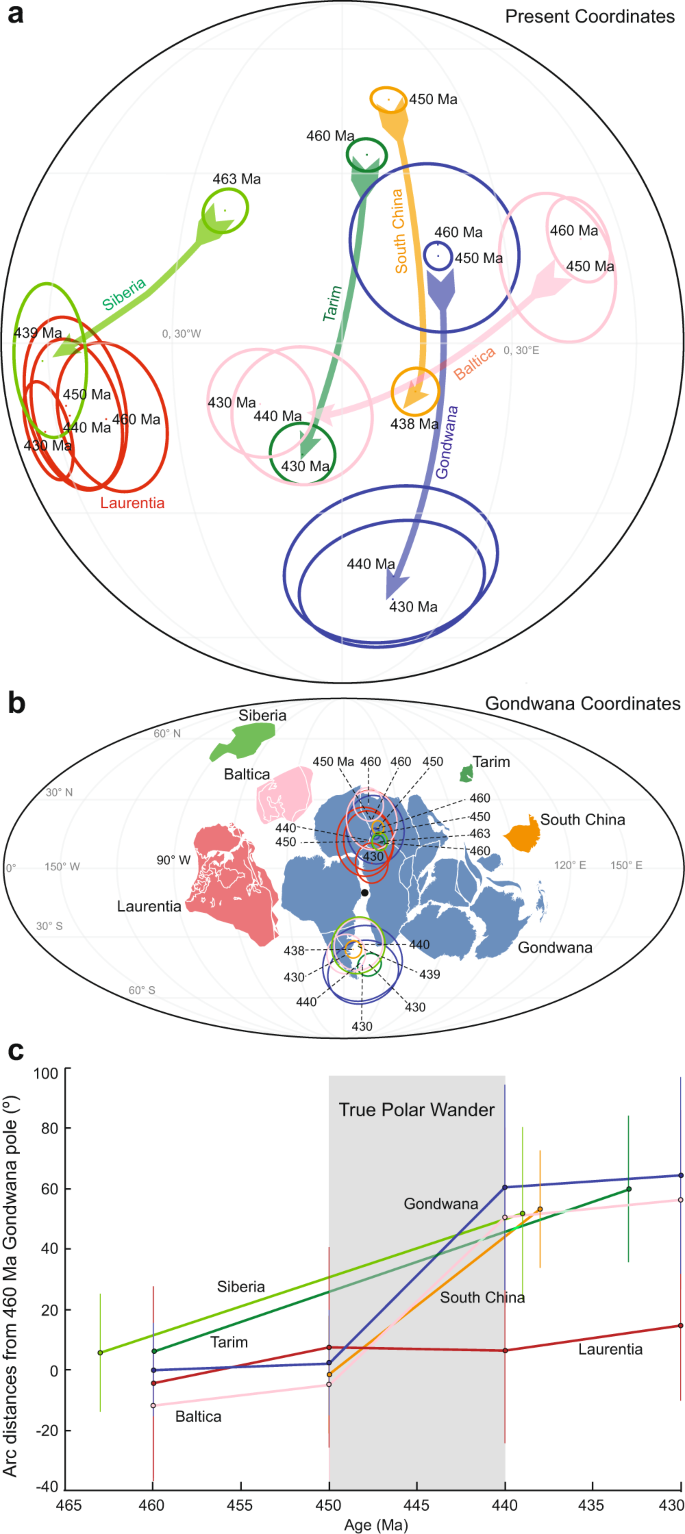
a Global palaeomagnetic poles for 460–430 Ma from South China, Tarim, Siberia, Baltica, Laurentia, and Gondwana. Apparent polar wander (APW) paths all exhibit (except Laurentia) large shifts between ca. 450–440 Ma. Poles shown in present-day coordinates. Pole information is listed in Supplementary Table 2 . b Poles rotated into the common reference frame of Gondwana illustrating the APW overlap. Black dot is a reference point in central Gondwana used for palaeolatitude estimates in Fig. 6e . c APW arc distances for all poles globally (in Gondwana reference frame of b ) relative to the 460 Ma Gondwana pole (as an arbitrary reference point before the hypothesized true polar wander event). See text for discussion of Laurentia. Vertical bars are intervals of 95% confidence. Plots in a and b were generated with GPlates 73 .
TPW could explain the large and synchronous dispersions of O–S palaeopoles globally. TPW is rate-limited by the ability of the viscous mantle to deform into a reoriented hydrostatic figure 45 , 46 . TPW can occur as fast as the fastest plate motion or even comparatively faster, particularly in more ancient times when the mantle was hotter, less viscous, and thus more deformable 46 , 47 . Numerical simulations suggest that a 40–50° amplitude TPW event can occur in ~10 Ma if the viscosity of lower mantle is 10 22 Pa s 46 . Presently lower mantle viscosity is about 3 × 10 22 Pa s 48 , while it may be 3 times lower at 450 Ma 47 . Hence, considering almost all continents sped up synchronously, we propose that during Late Ordovician, most likely after the middle Katian Stage but before the Silurian early Telychian Stage, a TPW event occurred. Furthermore, the fact that all the ~50° arc distances of APW are within statistical uncertainty of each other means that the data pass the global reproducibility test of TPW.
We note that palaeomagnetic poles from Laurentia during this time are characterized, in contrast, by much less APW, and almost essentially a stillstand (Fig. 3 ). At face value, one continent with a statistically different arc distance of APW compared with those of other continents does not invalidate the TPW hypothesis 49 . This point of caution is particularly relevant here because during this time Laurentia was an isolated plate with its own tectonic motion vector. In the Paleozoic, the Iapetus and Rheic oceans that existed in between Laurentia and West Gondwana rapidly expanded and vanished 12 , 34 , which certainly would have resulted in fast tectonic movements of Laurentia and may seem at odds with its small amount of APW. As the tectonic motion of Laurentia during the closure of the Iapetus would have been mostly opposite to its sense of motion due to TPW, the effect of TPW would be partially offset and thus should appear as a relative stillstand, where APW = plate motion + TPW. In this sense, as Laurentia would have undergone large tectonic motion during this time, its palaeomagnetic stillstand can only be reconciled if TPW in the opposite direction is invoked. Thus, the TPW event inferred from all other continents provides a convenient way to explain the prior paradox of a Laurentian APW stillstand during the closure of the Iapetus Ocean.
We should also note that, strictly speaking, Laurentia may not exhibit a total stillstand. The circles of 95% confidence of the youngest and oldest Laurentian poles (460 and 430 Ma) only very slightly overlap, and the results of an F test 50 demonstrate that the poles are distinct from each other at the 99% confidence level ( F = 10.6). This test indicates that the 18.9° ± 19.3° arc distance between the two O–S poles is statistically significant. Therefore, while the presumably considerable tectonic motion of Laurentia partially masks the ~50° TPW event, Laurentia nonetheless does indeed record a statistically significant portion of the TPW amplitude that, in reconstructed coordinates, is consistent with the sense of TPW rotation more clearly recorded on the other continents. Otherwise, this relative stillstand may be an artifact of the large age errors of these poles used for APW comparison 34 .
As defined as the migration of the maximum moment of inertia ( I max ) to align with Earth’s spin axis, TPW occurs as a rotation about an Euler pole controlled by the minimum moment of inertia ( I min ) that is equatorial and is therefore predicted to circumscribe a great-circle APW path. Identifying TPW as a great-circle APW path also assumes that plate motion of the continent relative to the mantle is negligible, the change in the orientation of the principal axes of non-hydrostatic moment of inertia is instantaneous, and those subsequently do not change at all. The similar amplitude and synchronicity of these five continents indicate their individual plate motions are negligible relative to the shared TPW motion. Numerical simulations indicate such a change in the orientation of the principal axes of non-hydrostatic moment of inertia can be completed within 10 Ma 46 . There is also a notable absence of poles in between the before/after poles recording the TPW shift (Fig. 3 ). These systematic gaps in the APW paths of all continents are consistent with the stroboscopic effect expected for TPW, which is a non-linear process that speeds up and slows down, thus rendering it less likely for rocks to form (making them available for palaeomagnetic sampling) during the peak rate of TPW in the middle of the event. A simple simulation (Methods) demonstrates that it is 20 times less likely to sample TPW “in action” than the endpoints largely before/after the TPW event (Fig. 4 ). This inherent bias can explain why the O–S TPW event is sampled exclusively by endpoints for all continents. We therefore confirm and refine the original proposal 27 of a large amplitude ~50° TPW event occurring across the O–S boundary.
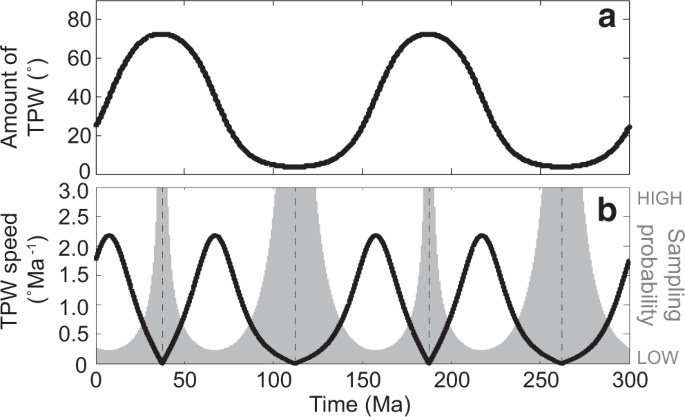
a True polar wander (TPW) angle as a function of time with an initial condition of 25°. b TPW speed (black line) and probability function (shaded gray) as a function of time.
Given this was a time of major plate tectonic reorganization in between assembly of megacontinent Gondwana and its larger supercontinent Pangaea 51 , there is no shortage of potential sources of subduction-related mass anomalies that might have provided the excitation for the large-scale TPW event across the O–S boundary. The Australian Tasmanides, the Laurentian Appalachians, and the Baltic Caledonides were all active at this time; however, provided their positions relatively close the TPW axis ( I min ), their influence on Earth’s rotation would have been dampened compared to mass anomalies elsewhere. In contrast, both the Proto-Tethyan and Terra Australis subduction systems on either side of Gondwana were ~90° away from I min and thus in the plane of TPW containing I max and I int would have been ideally positioned relative to Earth’s prolate non-hydrostatic figure to have excited large-scale TPW.
In the Late Ordovician, the Proto-Tethyan system experienced a fundamental shift from subduction to collision 52 . Both the timing (pre-TPW) and the sense of this change in slab dynamics—with the foundering oceanic slab likely ponding at the mantle transition zone, thus causing a positive anomaly in the geoid kernel driving TPW for this region equatorward 53 —are consistent with the observed palaeogeographic shift of the Tethyan subduction zone from mid-latitudes into the tropics (Fig. 5 ). Also, in the Terra Australis system on the other side of Gondwana, an intriguing coincidence is that the new position of the South Pole (post-TPW) becomes centered on the Antarctica–South America segment of the subduction system (Fig. 5 ) that experienced a dramatic shift from negative to positive hafnium isotopes at this age 54 . Such a shift due to increased mantle-derived magmatism in the arc indicates slab retreat, which can occur before slab break-off as a slab meets resistance to subduction after impinging the mantle transition zone 55 . Because of the time lag between slab subduction in the upper mantle and its penetration into the lower mantle, a dramatic slab avalanche from the upper into the lower mantle after stagnation at the mantle transition zone could thus conveniently explain the new pole position assumed in the Silurian as the geodynamic change in the Terra Australis would have driven TPW for this region poleward 53 . Thus, the dramatic changes in slab dynamics of both subduction systems on either side of Gondwana could have contributed to the collective forcing behind the largest TPW event in the past 500 million years.
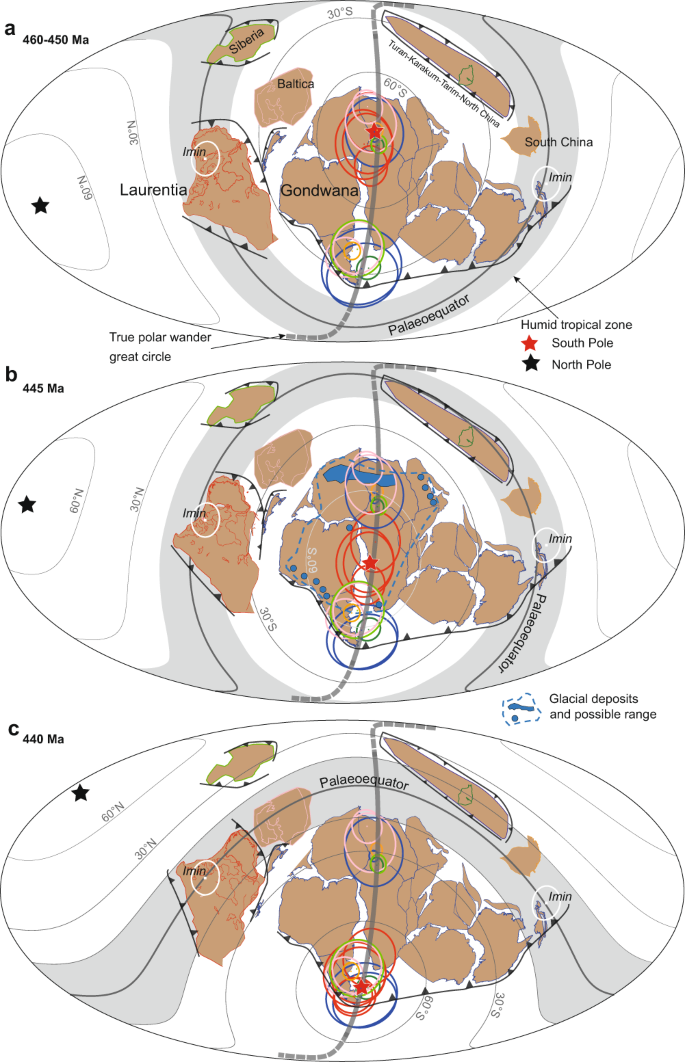
Reconstructions for: ( a ) 460–450 Ma, ( b ) 445 Ma, and ( c ) 440 Ma. Palaeomagnetic poles are color-coded as in Fig. 3a . I min , minimum moment of inertia (equatorial true polar wander axis of rotation). Palaeomagnetic poles (and associated continents) of each age are rotated to coincide with the South Pole, although a 5–10° range of flexibility is occasional adopted as is common praxis in ancient palaeomagnetic reconstructions. The latitudinal band between 15° north and south of the Equator is assigned as the humid tropical zone with intensive chemical weathering. Maps are shown in Mollweide projection. For better displaying their distribution, we fixed the continents and rotate the Mollweide projection to fit the Palaeo-south pole. All plots were generated with GPlates 73 .
It is also possible that the waxing and waning of ice sheets across Gondwana contributed to the mass anomalies driving O–S TPW, or there was some feedback between TPW and glaciation. In particular, there is a migration of glacial centers from northern Africa to southern Africa–South America, where glacial and periglacial strata in the former region are predominantly Ordovician and those in the latter neighboring regions are predominantly latest Ordovician or Silurian 15 . That is, the mass load associated with incipient Ordovician glaciation applied in northern Africa could have been driven to the equator by TPW, causing southern Africa–South America to move to the pole and thus moving the glacial center there in the earliest Silurian (Fig. 5 ). This hypothesis, by extension, would also predict ensuing oscillatory Silurian–Devonian TPW back in the direction of northern Africa (in order to drive the glacial center in southern Africa–South America equatorward), which has indeed been previously hypothesized 27 , but the assessment of which is beyond the scope of our study on O–S TPW. In the Cenozoic, however, glaciation is typically regarded more as an effect of TPW rather than a cause of it 56 , as the amplitude of glacially induced TPW is smaller than TPW driven by mass reorganizations in the mantle 56 . Nevertheless, given the larger size of the Paleozoic pan-Gondwanan ice sheet, and thus its presumably larger mass load, glacial loading deserves further investigation for potentially driving the O–S TPW event. If valid, such an interpretation—the incipient glacial load causing TPW, which then led to more severe glaciation as Gondwana became centered over the South Pole—presents a fascinating potential feedback between TPW and glaciation.
Palaeogeographic reconstructions based on true polar wander
Traditionally, the superposition of APW paths is used to reconstruct the configuration of different continents during time intervals of supercontinentality 57 . However, during times of plate tectonic reorganization in between supercontinents, this method cannot be used to reconstruct isolated continents that are in relative motion, which is most likely how the end-Ordovician world was kinematically configured 12 . Nonetheless, when APW is predominantly driven not by plate motion but by TPW, then the superposition of APW paths can be used to determine the relative positions of different continents whether they are united or isolated because the TPW motion is shared by all continents and thus provides a common global reference frame 26 , 58 . Such an APW comparison only requires a minimum of two poles from before and after the TPW event. Therefore, we can accurately reconstruct global paleogeography of the major continents across the O–S boundary by leveraging TPW.
To make our reconstructions, northwest Africa is fixed and all the other continents are rotated into northwest African coordinates (Euler rotation parameters listed in Supplementary Table 4 ). We first fitted a great circle to the palaeopoles from Gondwana, of which northwest Africa is a part (Fig. 5a ). Poles from all the other continents were then rotated to overlap the Gondwanan poles at their corresponding ages. The TPW-based reconstructions constrain the relative positions of all these continents during 460–440 Ma, not only including palaeolatitude constraints, but also commonly unconstrained relative palaeolongitude. As mentioned, the essentially opposite tectonic motion of Laurentia effectively cancels out some of the TPW rotation for Laurentia, therefore its position relative to other continents changes over time.
Three high-resolution, TPW-based palaeogeographic reconstructions are provided at 460–450, 445, and 440 Ma (Fig. 5 ). The 445 Ma reconstruction is an interpolated position between 450 and 440 Ma. Subduction zones and the evolution of Avalonia is simplified from Cocks and Torsvik 12 . A salient difference between our reconstructions and previous ones 5 , 6 , 8 , 12 , 59 is that Gondwana rapidly swept over the South Pole (Figs. 5 , 6e ). Meanwhile, during 460–450 Ma, the Niger–Chad zone was located at the South Pole rather than the Morocco–Algeria zone (Fig. 5a ). At 460–450 Ma, Gondwana was distributed from the South Pole to the Equator, with the majority of the landmass located at high-to-mid latitudes (Fig. 5a ). Laurentia straddled the equator, with its east coast (present coordinates) outside of the tropics. The positions of Baltica and Siberia are similar to previous reconstructions 5 , 6 , 12 , 59 . Constrained using the APW path of Tarim, Turan–Karakum–Tarim–North China 60 is constrained to a position between South China and Siberia. Most of South China was in the tropics, which is consistent with the palaeoequatorial setting suggested by the mega-nodular limestone, a time-specific carbonate facies 61 .
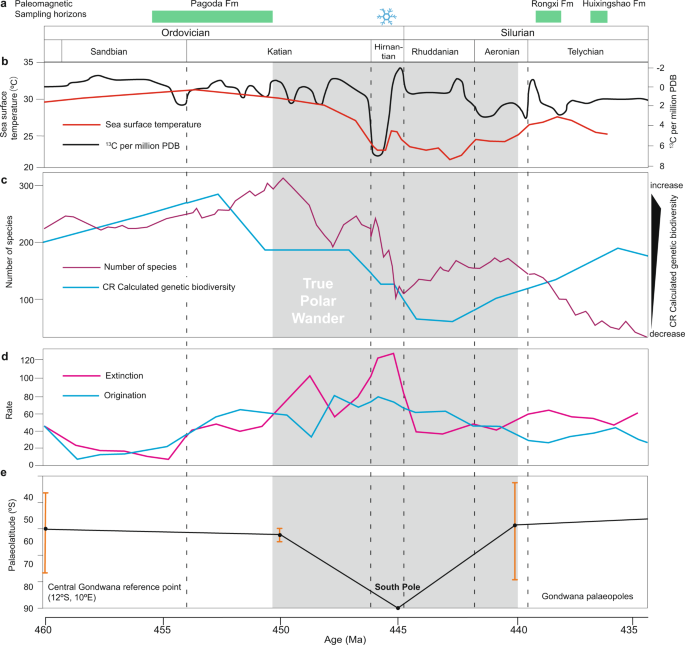
a Temporal distribution of the palaeomagnetic sampling horizons by Formation (Fm) from South China. Snowflake above the Hirnantian stage indicates the age of short, sharp glacial advance 3 . b δ 13 C record (black) and sea surface temperature variation (red) from Rasmussen et al. 7 c Biodiversity during the Sanbian–Telychian stages from Deng et al. 11 (purple line) and Rasmussen et al. 7 (blue line). CR capture–recapture modeling. d Rates of origination (blue) and extinction (magenta) with 1 million year age binning from Deng et al. 11 . e Palaeolatitudinal variation of a reference point in central Gondwana (12°S, 10°E) calculated by using 460–430 Ma Gondwana palaeopoles listed in Supplementary Table 2 . Note that the 430 Ma palaeolatitude is not displayed. Orange vertical bars are intervals of 95% confidence.
After 450 Ma, TPW initiated a dramatic change in palaeogeography. At 445 Ma, in the middle of the TPW event, northern Africa moved off the South Pole, where it was replaced by southern Africa and South America (Fig. 5b ). (In terms of tectonic motions, Laurentia moved closer to Baltica, but farther from Gondwana because of the fast opening of the Rheic Ocean.) During the TPW event, Baltica and Avalonia moved into low latitudes, and Siberia, Turan–Karakum–Tarim–North China, and South China ended up straddling the equator and were nearly all located within the tropics (Fig. 5b ). After the TPW event was over by 440 Ma (the Silurian), northern Africa and Arabia occupied low latitudes, while South America and southern Africa were located around the South Pole (Figs. 5 c, 6e ). Siberia and Turan–Karakum–Tarim–North China all moved out of the tropics, while Baltica moved into the tropics and South China moved into the tropics of the northern hemisphere. By the Silurian, more continents were positioned at mid-to-low latitudes (more than ~14,000,000 km 2 ; Fig. 5c ) than before (Fig. 5a, b ).
Having verified and refined the existence of a large-scale TPW rotation across the O–S boundary and reconstructed the associated rapid continental motions more precisely than ever before, we consider the potential impacts of such an extreme palaeogeographic disruption on the variegated changes to Earth’s surface conditions during this enigmatic time of transition. One unique puzzle of end-Ordovician global change is the occurrence of severe glaciation. Continental configuration is known to play an important role in setting the climate state on both long and short time scales 2 , 62 , so any changes in paleogeography due to TPW should also be critically considered. Phanerozoic glaciations have been generally correlated with the occurrences of arc-continent collisions distributed within the humid tropical zone, which serves to lower global temperatures by increasing silicate weathering and consuming CO 2 , an atmospheric greenhouse gas 2 , 62 .
Reliable evidence of O–S glacial deposits from Gondwana that was positioned near the South Pole at the time is mostly found during the Hirnantian stage, with only sporadic cases reported before and after 63 . The latest Katian and early Silurian glaciogenic sediments occurring before and after the Hirnantian, respectively, found in Niger and South America argue for a medium-to-large-scale glacial interval, if using previous paleogeography 5 , 6 , 8 , 12 , 59 . However, no other sectors of Gondwana record glaciogenic sediments during these time intervals. Furthermore, cyclostratigraphic analysis also indicates the main glaciation initiated in the early Hirnantian stage 64 . In our reconstruction of the latest Katian, Niger was close to the South Pole (Fig. 5a ), where glaciation is most likely to develop as it is the coldest place on Earth because incoming solar radiation is reduced by the high angle of incidence at high latitudes, as evidenced in the current polar ice caps of Antarctica and Greenland. Meanwhile, during the early Silurian, South America moved over the South Pole (Fig. 5c ) and glaciation, as expected, occurred there again. Therefore, our reconstructions match the migration of glacial centers across Gondwana quite well 15 , 16 , 63 , which in turn independently validates our proposal of O–S TPW. In particular, the fact that the short, sharp Hirnantian glaciation 3 is shown to have occurred precisely in the middle of the TPW event—as Gondwana swept over the South Pole (Figs. 5 , 6e ) and its ice sheet presumably should have expanded to its largest size for a very brief time interval—indicates that TPW is a previously unrecognized major factor in the total causal nexus explaining the Hirnantian glaciation, as for the Cenozoic northern hemisphere glaciation 56 , 65 .
We next consider how TPW interacted with other factors previously thought to control Late Ordovician early Silurian glaciation. Volcanic eruption 4 , 7 , plant and large phytoplankton evolution 9 , 10 , and silicate weathering 2 , 62 , 66 have all been proposed to explain the extreme climate change across the O–S boundary. However, previous palaeogeographic constraints limited the accuracy of such interpretations 6 , 14 , 59 . Our reconstructions demonstrate that after 450 Ma, TPW placed Siberia, Turan–Karakum–Tarim–North China, and South China entirely into the tropics; in addition, a large portion of Gondwana was moved down from high- to mid-latitudes (Fig. 5b ). All these palaeogeographic changes thus favor the observed intensification of silicate weathering helping drive cooling. These palaeogeographic conditions resulted in not only the Hirnantian glaciation, but also the marked positive Hirnantian carbon isotope excursion (Fig. 6b ) due to the increased fraction of organic carbon burial resulting from the preponderance of tropical continental margins (Fig. 5b ) analogous to the modern Amazon River. Previous studies suggest that arc-continent collisions within the humid tropical zone set Earth’s climate state to first order during the Phanerozoic Eon 2 . After the peak glaciation and positive carbon isotope excursion, a large portion of the arc-continent collisions of Laurentia, Siberia, and Turan–Karakum–Tarim–North China moved out of the tropics (Fig. 5c ), thereby silicate weathering plummeted causing deglaciation and carbon cycle recovery (Fig. 6 ).
Although there are myriad ways in which TPW may indirectly affect biodiversity through environmental change 26 , a direct link has also been proposed through a true polar wander–latitudinal diversity gradient (TPW–LDG) theory 18 , 21 . It suggests that continents shifting equatorward, i.e., moving into the LDG, would experience enhanced origination and hence diversity increase, while those shifting poleward, i.e., moving out of the LDG, would experience enhanced extinction and hence diversity decrease. This quadrature pattern of TPW effects on diversity is similar to those predicted for relative sea-level change during TPW 25 , which can further amplify the anticipated diversity changes through sea-level-related artifacts, e.g., continental margins moving equatorward should experience elevated origination (though TPW–LDG theory), and additionally, fossils of these new species are more likely to be preserved due to the concomitant transgression in sea level 21 . Recently reported high-resolution biodiversity records 1 , 7 , 11 suggest a protracted two- or three-phase extinction lasting from the Katian stage to the Hirnantian stage (Fig. 6c, d ), rather than a two-pulse extinction limited to the Hirnantian 5 . This modified extinction pattern may indicate that previous kill mechanisms proposed to fit the two-pulse extinction situation have become at least partially invalidated or weakened 59 .
Our reconstructions demonstrate that during 450–445 Ma, TPW moved southern Gondwana poleward and northern Gondwana equatorward (Fig. 5 ), with more area of Gondwana on average shifting poleward (Fig. 5a, b ). This observation, according to TPW–LDG theory, would cause both enhanced origination and extinction, but with extinction overwhelming origination. This palaeogeographic prediction appears to be supported by the fossil record 11 (Fig. 6d ). After that, a radiation phase (Fig. 6c, d ) should correspond with the continuing equatorward shift of Gondwana, Baltica, and Turan–Karakum–Tarim–North China until the early Hirnantian. Thus, during the fleeting Hirnantian stage, both the increased tropical weathering of arc-continent collisions triggered glaciation and the further poleward shifting of Siberia, Turan–Karakum–Tarim–North China, South China and south and east Gondwana, caused the second severe extinction (Fig. 6c, d ). Paleogeography after the TPW event also favored plant colonization as a majority of continents became located at low-to-mid latitudes (Fig. 5c ), which is supported by the positive carbon isotope signal (Fig. 6b ) and oxygen rise during this time 7 . Overall, the proposed TPW and the palaeogeographic reorganization resulting from the ~50˚ reorientation provide a simple and basic mechanism for the dramatic environmental changes of the end Ordovician early Silurian. These connections reflect the intimate coupling between the evolution of Earth’s spheres: TPW is induced by changes of subducting slabs in the mantle; in turn, TPW resulted in palaeogeographic changes that influenced Earth’s hydrosphere, cryosphere, and biosphere.
Our sampling sections are in Xiushan County, east Chongqing, China (Supplementary Figs. 1 , 2 ). Silurian strata in this area were folded during the middle Mesozoic (Jurassic–Cretaceous) 67 . Ascending in stratigraphic order, the Silurian strata consist of the Llandovery Longmaxi Formation (Fm) black shale, the Xiaoheba Fm green siltstone, the Rongxi Fm red beds, the Xiushan Fm siltstone, the Huixingshao Fm red beds, and the Ludlow-Pridoli Xiaoxi Fm siltstone with some red beds (Supplementary Figs. 1 , 2 ). There are disconformities between the Silurian strata and both its overlying Devonian and underlying Ordovician strata (Supplementary Figs. 1 , 2 ).
As previous studies 32 , 35 had intensively sampled the Rongxi Fm (Supplementary Fig. 1 ), we conducted our palaeomagnetic study on the previously sparsely sampled Huixingshao Fm at three sections (Supplementary Figs. 1 , 2 ). The section Yongdong (SY) is on the west limb of a steeply dipping syncline (GPS: 28.610°N, 109.157°E; Supplementary Fig. 2 ). Six sites about 104 samples are collected here. The sections Tianlu and Kapeng (ST and SK) are on the east limb of the same syncline (ST GPS: 28.551°N, 109.162°E; SK GPS: 28.631°N, 109.287°E; Supplementary Fig. 2 ). One site (10 samples) and four sites (43 samples) were collected from the ST and SK sections, respectively. All samples were collected with a portable gasoline-powered drill and oriented with a magnetic compass.
Palemagnetism and rock magnetism
All samples were cut into at least one standard specimen (height = 2.3 cm, diameter = 2.54 cm). Natural remanent magnetization (NRM) was firstly measured. Then, all specimens were subjected to stepwise thermal demagnetization using an ASC-TD48 oven, and remanent magnetizations were measured using a 2G-755 cryogenic superconducting magnetometer housed in a magnetically shielded room. Typically, demagnetization was applied in steps of 10–100 °C, starting at 100 °C and going up to 670 °C. Backfield demagnetization curves of representative specimens, with a 2 T saturation field followed by a progressively larger filed in opposite direction, were conducted on the VSM 8600 series (Lake Shore Cryotronics, Inc.). Susceptibility temperature ( κ-T ) experiments, heated and cooled between 30–700 °C, were measured in air by using the KLY4 Kappa bridge (AGICO) devices. All experiments were done at the Key Laboratory of Paleomagnetism and Reconstruction, Ministry of Natural Resources, Beijing.
Probability of sampling true polar wander
For any quantity, φ , that varies as a function of time, the probability density function (PDF) describes the relative amount of time spent at each value of φ or, alternatively, the likelihood that a particular value of φ will be sampled. For TPW, we let φ(t) be the angular separation of the rotation axis relative to a fixed geographic axis (TPW angle), or the angular distance from one estimate of the location of the spin axis to some later estimate. An un-normalized PDF for φ(t) is then given by
so that the probability of sampling φ within a given angular interval is
where α is a constant chosen so that the total probability P (0° < φ < 90°) equals 1. Equation ( 1 ) shows that the likelihood that φ is sampled is exactly inversely proportional to the TPW speed, | dφ/dt | . This straightforward relationship between TPW sampling probability (PDF) and TPW speed shows that faster instantaneous TPW speeds are prone to be undersampled. Technically speaking, the uphill battle to observe rapid TPW is nothing more than the age-old stroboscopic effect, or aliasing: the difficulty of studying rotating planets, reciprocating blades, oscillating fans, or vibrating strings with discrete data; temporal undersampling is a major hurdle for understanding geologic processes 68 and TPW is not an exception.
We now demonstrate an example of the undersampling problem with a simple modeled TPW excursion. In the following example, we use the simple analytical framework of Tsai and Stevenson 69 to describe the TPW due to a chosen moment of inertia tensor variation. In this formulation, the Liouville equation for a viscoelastic planet is analytically solved for a given perturbation of the moment of inertia tensor, following Munk and MacDonald 70 , to obtain the TPW angle. For simplicity, we chose a moment of inertia variation that is sinusoidal with a period of 150 Ma, with an average viscosity of 3 × 10 22 Pa s, and an amplitude of 10 −5 C (where C is the Earth’s moment of inertia). This variation is chosen to very roughly approximate the observed TPW described in the next section. Equation (12) of Tsai and Stevenson 69 then yields the TPW angle as a function of time, φ(t) , which is plotted in Fig. 4a for an arbitrary chosen initial condition. The associated TPW speed (black line) and the PDF (shaded gray) for this TPW curve are plotted as a function of time in Fig. 4b . As shown, the maximum instantaneous TPW speed is about 2.2° Ma −1 (24 cm yr −1 ) and is associated with a minimum in the PDF, showing that it is the least probable value to be observed if one randomly samples the distribution. One can also compare the probability of sampling within a finite range by using Eq. ( 2 ), or by simply reading time intervals from Fig. 4a . For example, the values of φ in the range 4° < φ < 9° represent ~32% of all measurements whereas a similar 5° range 40° < φ < 45° represents only about 1.5% of all measurements. For this example, then, it is about 20 times more likely to sample within the first range of φ (low instantaneous TPW speed) compared with the second range (maximum instantaneous TPW speed).
Data availability
The palaeomagnetic data generated in this study, including the magnetometer measurements and backfield demagnetization data, have been deposited in the Open Science Framework database ( https://osf.io/z2cds/ ). Palaeomagnetic statistical data, palaeopoles and Euler parameters used in this study are provided in the Supplementary information.
Fan, J. et al. A high-resolution summary of Cambrian to Early Triassic marine invertebrate biodiversity. Science 367 , 272–277 (2020).
Article ADS CAS Google Scholar
Macdonald, F. A., Swanson-Hysell, N. L., Park, Y., Lisiecki, L. & Jagoutz, O. Arc-continent collisions in the tropics set Earth’s climate state. Science 364 , 181–184 (2019).
Finnegan, S. et al. The magnitude and duration of Late Ordovician–early Silurian glaciation. Science 331 , 903–906 (2011).
Bond, D. P. G. & Grasby, S. E. Late Ordovician mass extinction caused by volcanism, warming, and anoxia, not cooling and glaciation. Geology 48 , 777–781 (2020).
Harper, D. A. T., Hammarlund, E. U. & Rasmussen, C. M. Ø. End Ordovician extinctions: a coincidence of causes. Gondwana Res. 25 , 1294–1307 (2014).
Article ADS Google Scholar
Longman, J., Mills, B. J. W., Manners, H. R., Gernon, T. M. & Palmer, M. R. Late Ordovician climate change and extinctions driven by elevated volcanic nutrient supply. Nat. Geosci. 14 , 924–929 (2021).
Rasmussen, C. M. Ø., Kröger, B., Nielsen, M. L. & Colmenar, J. Cascading trend of Early Paleozoic marine radiations paused by Late Ordovician extinctions. Proc. Natl Acad. Sci. USA 116 , 7207–7213 (2019).
Saupe, E. E. et al. Extinction intensity during Ordovician and Cenozoic glaciations explained by cooling and palaeogeography. Nat. Geosci. 13 , 65–70 (2020).
Lenton, T. M., Crouch, M., Johnson, M., Pires, N. & Dolan, L. First plants cooled the Ordovician. Nat. Geosci. 5 , 86–89 (2012).
Shen, J. et al. Improved efficiency of the biological pump as a trigger for the Late Ordovician glaciation. Nat. Geosci. 11 , 510–514 (2018).
Deng, Y. et al. Timing and patterns of the great Ordovician biodiversification event and Late Ordovician mass extinction: perspectives from South China. Earth-Sci. Rev. 220 , 103743 (2021).
Article Google Scholar
Cocks, L. R. M. & Torsvik, T. H. Ordovician palaeogeography and climate change. Gondwana Res. 100 , 53–72 (2021).
Merdith, A. S. et al. Extending full-plate tectonic models into deep time: linking the Neoproterozoic and the Phanerozoic. Earth-Sci. Rev. 214 , 103477 (2021).
Mills, B. J. W. et al. Modelling the long-term carbon cycle, atmospheric CO2, and Earth surface temperature from late Neoproterozoic to present day. Gondwana Res. 67 , 172–186 (2019).
Caputo, M. V. & Crowell, J. C. Migration of glacial centers across Gondwana during Paleozoic Era. Geol. Soc. Am. Bull. 96 , 1020 (1985).
2.0.CO;2" data-track-item_id="10.1130/0016-7606(1985)96 2.0.CO;2" data-track-value="article reference" data-track-action="article reference" href="https://doi.org/10.1130%2F0016-7606%281985%2996%3C1020%3AMOGCAG%3E2.0.CO%3B2" aria-label="Article reference 15" data-doi="10.1130/0016-7606(1985)96 2.0.CO;2">Article ADS Google Scholar
López-Gamundí, O. R. & Buatois, L. A. Introduction: Late Paleozoic glacial events and postglacial transgressions in Gondwana. Geol. Soc. Am . https://doi.org/10.1130/2010.2468(00) (2010).
Kirschvink, J. L., Ripperdan, R. L. & Evans, D. A. Evidence for a large-scale reorganization of early cambrian continental masses by inertial interchange true polar wander. Science 277 , 541–545 (1997).
Article CAS Google Scholar
Kirschvink, J. L. & Raub, T. D. A methane fuse for the Cambrian explosion: carbon cycles and true polar wander. Comptes Rendus Geosci. 335 , 65–78 (2003).
Mitchell, R. N., Evans, D. A. D. & Kilian, T. M. Rapid early Cambrian rotation of Gondwana. Geology 38 , 755–758 (2010).
Mitchell, R. N. et al. Sutton hotspot: resolving Ediacaran-Cambrian tectonics and true polar wander for Laurentia. Am. J. Sci. 311 , 651–663 (2011).
Mitchell, R. N., Raub, T. D., Silva, S. C. & Kirschvink, J. L. Was the Cambrian explosion both an effect and an artifact of true polar wander? Am. J. Sci. 315 , 945–957 (2015).
Robert, B. et al. Constraints on the Ediacaran inertial interchange true polar wander hypothesis: a new paleomagnetic study in Morocco (West African Craton). Precambrian Res. 295 , 90–116 (2017).
Antonio, P. Y. J., Trindade, R. I. F., Giacomini, B., Brandt, D. & Tohver, E. New high-quality paleomagnetic data from the Borborema Province (NE Brazil): refinement of the APW path of Gondwana in the Early Cambrian. Precambrian Res. 360 , 106243 (2021).
Maloof, A. C. et al. Combined paleomagnetic, isotopic, and stratigraphic evidence for true polar wander from the Neoproterozoic Akademikerbreen Group, Svalbard, Norway. Geol. Soc. Am. Bull . https://doi.org/10.1130/B25892.1 (2006).
Mound, J. E. & Mitrovica, J. X. True polar wander as a mechanism for second-order sea-level variation. Science 279 , 534–537 (1998).
Raub, T. D., Kirschvink, J. L. & Evans, D. A. D. in Treatise on Geophysics 511–530 (Elsevier, 2007).
Van der Voo, R. True polar wander during the middle Paleozoic? Earth Planet. Sci. Lett. 122 , 239–243 (1994).
Mitchell, R. N. True polar wander and supercontinent cycles: implications for lithospheric elasticity and the triaxial earth. Am. J. Sci. 314 , 966–979 (2014).
Piper, J. A. ∼ 90° Late Silurian–Early Devonian apparent polar wander loop: the latest inertial interchange of planet earth? Earth Planet. Sci. Lett. 250 , 345–357 (2006).
Han, Z., Yang, Z., Tong, Y. & Jing, X. New paleomagnetic results from Late Ordovician rocks of the Yangtze Block, South China, and their paleogeographic implications. J. Geophys. Res. Solid Earth 120 , 4759–4772 (2015).
Huang, B., Piper, J. D. A., Sun, L. & Zhao, Q. New paleomagnetic results for Ordovician and Silurian rocks of the Tarim Block, Northwest China and their paleogeographic implications. Tectonophysics 755 , 91–108 (2019).
Huang, K., Opdyke, N. D. & Zhu, R. Further paleomagnetic results from the Silurian of the Yangtze Block and their implications. Earth Planet. Sci. Lett. 175 , 191–202 (2000).
Smethurst, M. A., Khramov, A. N. & Torsvik, T. H. The Neoproterozoic and Palaeozoic palaeomagnetic data for the Siberian Platform: from Rodinia to Pangea. Earth-Sci. Rev. 43 , 1–24 (1998).
Wu, L. et al. The amalgamation of Pangea: paleomagnetic and geological observations revisited. GSA Bull. 133 , 625–646 (2021).
Opdyke, N. D., Huang, K., Xu, G., Zhang, W. Y. & Kent, D. V. Paleomagnetic results from the Silurian of the Yangtze paraplatform. Tectonophysics 139 , 123–132 (1987).
Meert, J. G. et al. The magnificent seven: a proposal for modest revision of the quality index. Tectonophysics 790 , 228549 (2020).
Rong, J. et al. Silurian integrative stratigraphy and timescale of China. Sci. China Earth Sci. 62 , 89–111 (2019).
Chen, Z. et al. Age of the Silurian lower red beds in South China: stratigraphical evidence from the Sanbaiti Section. J. Earth Sci. 32 , 524–533 (2021).
Cai, J., Zhao, W. & Zhu, M. Subdivision and age of the Silurian fish-bearing Kuanti formation in Qujing, Yunnan Province. Vertebr. Palasiat. 58 , 249–266 (2020).
Google Scholar
McFadden, P. L. A new fold test for palaeomagnetic studies. Geophys. J. Int 103 , 163–169 (1990).
Shu, L. et al. Neoproterozoic plate tectonic process and Phanerozoic geodynamic evolution of the South China Block. Earth-Sci. Rev. 216 , 103596 (2021).
Evans, D. A. D. Proterozoic low orbital obliquity and axial-dipolar geomagnetic field from evaporite palaeolatitudes. Nature 444 , 51–55 (2006).
Abrajevitch, A. & Van der Voo, R. Incompatible Ediacaran paleomagnetic directions suggest an equatorial geomagnetic dipole hypothesis. Earth Planet. Sci. Lett. 293 , 164–170 (2010).
Torsvik, T. H. et al. Phanerozoic polar wander, palaeogeography and dynamics. Earth-Sci. Rev. 114 , 325–368 (2012).
Tsai, V. C. & Stevenson, D. J. Theoretical constraints on true polar wander. J. Geophys. Res. Solid Earth https://doi.org/10.1029/2005JB003923 (2007).
Creveling, J. R., Mitrovica, J. X., Chan, N.-H., Latychev, K. & Matsuyama, I. Mechanisms for oscillatory true polar wander. Nature 491 , 244–248 (2012).
Crowley, J. W., Gérault, M. & O’Connell, R. J. On the relative influence of heat and water transport on planetary dynamics. Earth Planet. Sci. Lett. 310 , 380–388 (2011).
Mitrovica, J. X. & Forte, A. M. A new inference of mantle viscosity based upon joint inversion of convection and glacial isostatic adjustment data. Earth Planet. Sci. Lett. 225 , 177–189 (2004).
Evans, D. True polar wander and supercontinents. Tectonophysics https://doi.org/10.1016/S0040-1951(02)00642-X (2003).
Butler, R. Paleomagnetism: Magnetic Domains to Geologic Terranes (Electronic Edition, 1998).
Wang, C., Mitchell, R. N., Murphy, J. B., Peng, P. & Spencer, C. J. The role of megacontinents in the supercontinent cycle. Geology 49 , 402–406 (2021).
Wang, C. et al. Age, nature, and origin of Ordovician Zhibenshan granite from the Baoshan terrane in the Sanjiang region and its significance for understanding Proto-Tethys evolution. Int. Geol. Rev. 57 , 1922–1939 (2015).
Steinberger, B., Seidel, M. & Torsvik, T. H. Limited true polar wander as evidence that Earth’s nonhydrostatic shape is persistently triaxial. Geophys. Res. Lett. 44 , 827–834 (2017).
Nelson, D. A. & Cottle, J. M. Long-term geochemical and geodynamic segmentation of the Paleo-Pacific margin of Gondwana: Insight from the Antarctic and adjacent sectors: Segmentation of the Gondwana margin. Tectonics 36 , 3229–3247 (2017).
Nakakuki, T. & Mura, E. Dynamics of slab rollback and induced back-arc basin formation. Earth Planet. Sci. Lett. 361 , 287–297 (2013).
Woodworth, D. & Gordon, R. G. Paleolatitude of the Hawaiian Hot Spot Since 48 Ma: evidence for a Mid‐Cenozoic True Polar Stillstand followed by Late Cenozoic True polar wander coincident with Northern Hemisphere glaciation. Geophys. Res. Lett . https://doi.org/10.1029/2018GL080787 (2018).
Mitchell, R. N. et al. The supercontinent cycle. Nat. Rev. Earth Environ. 2 , 358–374 (2021).
Steinberger, B. & Torsvik, T. H. Absolute plate motions and true polar wander in the absence of hotspot tracks. Nature 452 , 620–623 (2008).
Pohl, A. et al. Vertical decoupling in Late Ordovician anoxia due to reorganization of ocean circulation. Nat. Geosci. 14 , 868–873 (2021).
Zuza, A. V. & Yin, A. Balkatach hypothesis: a new model for the evolution of the Pacific, Tethyan, and Paleo-Asian oceanic domains. Geosphere 13 , 1664–1712 (2017).
Zhan, R. et al. Meganodular limestone of the Pagoda formation: a time-specific carbonate facies in the Upper Ordovician of South China. Palaeogeogr. Palaeoclimatol. Palaeoecol. 448 , 349–362 (2016).
Swanson-Hysell, N. L. & Macdonald, F. A. Tropical weathering of the Taconic orogeny as a driver for Ordovician cooling. Geology https://doi.org/10.1130/G38985.1 (2017).
Wang, G., Zhan, R. & Percival, I. G. The end-Ordovician mass extinction: a single-pulse event? Earth-Sci. Rev. 192 , 15–33 (2019).
Sinnesael, M. et al. Precession-driven climate cycles and time scale prior to the Hirnantian glacial maximum. Geology 49 , 1295–1300 (2021).
Daradich, A., Huybers, P., Mitrovica, J. X., Chan, N.-H. & Austermann, J. The influence of true polar wander on glacial inception in North America. Earth Planet. Sci. Lett. 461 , 96–104 (2017).
Kump, L. R. et al. A weathering hypothesis for glaciation at high atmospheric pCO2 during the Late Ordovician. Palaeogeogr. Palaeoclimatol. Palaeoecol. 152 , 173–187 (1999).
Liu, L. et al. Geometry and timing of Mesozoic deformation in the western part of the Xuefeng Tectonic Belt, South China: Implications for intra-continental deformation. J. Asian Earth Sci . 49 , 330–338 (2012).
Wiggert, J., Dickey, T. & Granata, T. The effect of temporal undersampling on primary production estimates. J. Geophys. Res. 99 , 3361 (1994).
Tsai, V. C. & Stevenson, D. J. Theoretical constraints on true polar wander. J. Geophys. Res. 112 , B05415 (2007).
ADS Google Scholar
Munk, W. & MacDonald, G. J. The Rotation of the Earth: A Geophysical Discussion (Cambridge University Press, 2009).
Jing, X., Yang, Z., Tong, Y., Wang, H. & Xu, Y. Identification of multiple magnetizations of the Ediacaran strata in South China. Geophys. J. Int 212 , 54–75 (2018).
Cogné, J. P. PaleoMac: A Macintosh TM application for treating paleomagnetic data and making plate reconstructions. Geochem. Geophys. Geosystems https://doi.org/10.1029/2001GC000227 (2003).
Müller, R. D. et al. GPlates: building a virtual earth through deep time. Geochem. Geophys. Geosystems 19 , 2243–2261 (2018).
Download references
Acknowledgements
We thank Nie Lei and Chen Yang of Chongqing Key Laboratory of Exogenic Mineralization and Mine Environment, Chongqing Institute of Geology and Mineral Resources for information about the sampling sections and assistance to the drafting of Supplementary Fig. 2 . Victor Tsai provided Fig. 4 depicting the probability of sampling TPW. This study was supported by the Natural Science Foundation of China (grants 91855216, 41230208, 42002208). X.J. was supported by the China Scholarship Council (no. 201808110036). R.M. was supported by the Natural Science Foundation of China (grant 41888101) and the Key Research Program of the Institute of Geology & Geophysics, CAS (grant IGGCAS-201905).
Author information
Authors and affiliations.
College of Resources, Environment and Tourism, Capital Normal University, Beijing, China
Xianqing Jing & Zhenyu Yang
State Key Laboratory of Lithospheric Evolution, Institute of Geology and Geophysics, Chinese Academy of Sciences, Beijing, China
Ross N. Mitchell & Bo Wan
Institute of Geomechanics, Chinese Academy of Geological Sciences, Beijing, China
Key Laboratory of Vertebrate Evolution and Human Origins of Chinese Academy of Sciences, Institute of Vertebrate Paleontology and Paleoanthropology, Chinese Academy of Sciences, Beijing, China
You can also search for this author in PubMed Google Scholar
Contributions
X.J., R.M., Z.Y. concept this research. X.J., Z.Y., M.Z., Y.T. conducted the field investigation and sampling. X.J. implemented all the thermal demagnetization and rock magnetic experiments. X.J., R.M., Z.Y., B.W. analyzed the data. Figures 1 l, 3 b, 5a–c , Supplementary Fig. 2 and Supplementary Fig. 5 were generated by X.J. Snowflake in Fig. 6a was generated by X.J. Map used in Supplementary Fig. 1 was generated by X.J. R.M. drew the Fig. 4 . Figures 3 , 5 , 6 were generated by X.J. and R.M. X.J., R.M., Z.Y. wrote the original draft. All authors contributed to the manuscript rewriting and editing.
Corresponding authors
Correspondence to Zhenyu Yang or Ross N. Mitchell .
Ethics declarations
Competing interests.
The authors declare no competing interests.
Peer review
Peer review information.
Nature Communications thanks Richard Gordon and the other, anonymous, reviewer(s) for their contribution to the peer review of this work. Peer reviewer reports are available.
Additional information
Publisher’s note Springer Nature remains neutral with regard to jurisdictional claims in published maps and institutional affiliations.
Supplementary information
Supplementary information, peer review file, rights and permissions.
Open Access This article is licensed under a Creative Commons Attribution 4.0 International License, which permits use, sharing, adaptation, distribution and reproduction in any medium or format, as long as you give appropriate credit to the original author(s) and the source, provide a link to the Creative Commons license, and indicate if changes were made. The images or other third party material in this article are included in the article’s Creative Commons license, unless indicated otherwise in a credit line to the material. If material is not included in the article’s Creative Commons license and your intended use is not permitted by statutory regulation or exceeds the permitted use, you will need to obtain permission directly from the copyright holder. To view a copy of this license, visit http://creativecommons.org/licenses/by/4.0/ .
Reprints and permissions
About this article
Cite this article.
Jing, X., Yang, Z., Mitchell, R.N. et al. Ordovician–Silurian true polar wander as a mechanism for severe glaciation and mass extinction. Nat Commun 13 , 7941 (2022). https://doi.org/10.1038/s41467-022-35609-3
Download citation
Received : 17 May 2022
Accepted : 13 December 2022
Published : 26 December 2022
DOI : https://doi.org/10.1038/s41467-022-35609-3
Share this article
Anyone you share the following link with will be able to read this content:
Sorry, a shareable link is not currently available for this article.
Provided by the Springer Nature SharedIt content-sharing initiative

This article is cited by
In-situ δ18o and 87sr/86sr proxies in an unconformable clastic unit at the ordovician–silurian transition.
- Chaewon Park
- Yungoo Song
- Youn-Joong Jeong
Scientific Reports (2023)
The influence of Tethyan evolution on changes of the Earth’s past environment
- Rixiang Zhu
Science China Earth Sciences (2023)
True polar wander in the Earth system
- Ross N. Mitchell
By submitting a comment you agree to abide by our Terms and Community Guidelines . If you find something abusive or that does not comply with our terms or guidelines please flag it as inappropriate.
Quick links
- Explore articles by subject
- Guide to authors
- Editorial policies
Sign up for the Nature Briefing newsletter — what matters in science, free to your inbox daily.

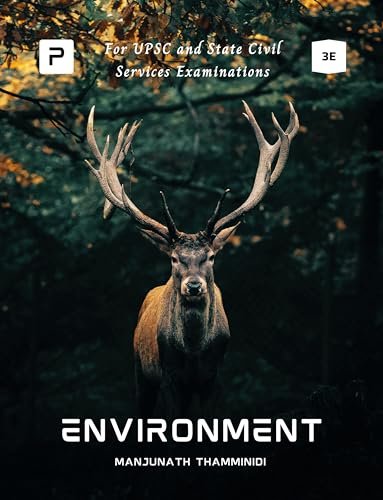
Discounts on PMF IAS Courses and PMF IAS PDFs ENDS Today! Hurry Up!
Continental Drift Theory: Evidences and Drawbacks, Tectonics
- December 16, 2019
- Dec 28, 2019
Subscribe to Never Miss an Important Update! Assured Discounts on New Products!
PMF IAS Telegram Channel | 53 MCQs in Prelims 2024 From PMF IAS
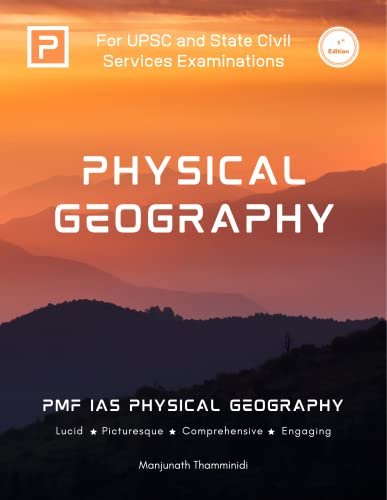
- During WW II, it was discovered that the ocean floor had some unique relief features like ridges, trenches, seamounts, shoals etc.
- Ridges and trenches gave insights into natural boundaries between various lithospheric plates (tectonic plates). These important discoveries led to the field of tectonics in geology.
- Tectonics is the scientific study of forces (convection currents in the mantle ) and processes (collisions of the lithospheric plates, folding, faulting, volcanism) that control the structure of the Earth’s crust and its evolution through time.
- It is basically about understanding the large-scale deformation of the lithosphere ( crust and upper mantle above asthenosphere ) and the forces that produce such deformation.
- It deals with the folding and faulting associated with mountain building; the large-scale, gradual upward and downward movements of the crust (epeirogenic movements); the growth and behaviour of old cores of continents known as cratons; and sudden horizontal displacements along faults.
Important concepts that tried to explain the tectonic processes
Continental drift theory (cdt).
- Continental drift refers to the movement of the continents relative to each other.
Polar wandering (similar to Continental Drift Theory)
- Polar wandering is the relative movement of the earth’s crust and upper mantle with respect to the rotational poles of the earth.
Seafloor Spreading Theory (SFST)
- Seafloor spreading describes the movement of oceanic plates relative to one another.
Plate Tectonics (PT)
- Plate tectonics is the movement of lithospheric plates relative to each other.
Convection Current Theory (CCT)
- Convection current theory forms the basis of SFST and PT. It explains the force behind plate movements.
Continental Drift Theory (Alfred Wegener, 1922)
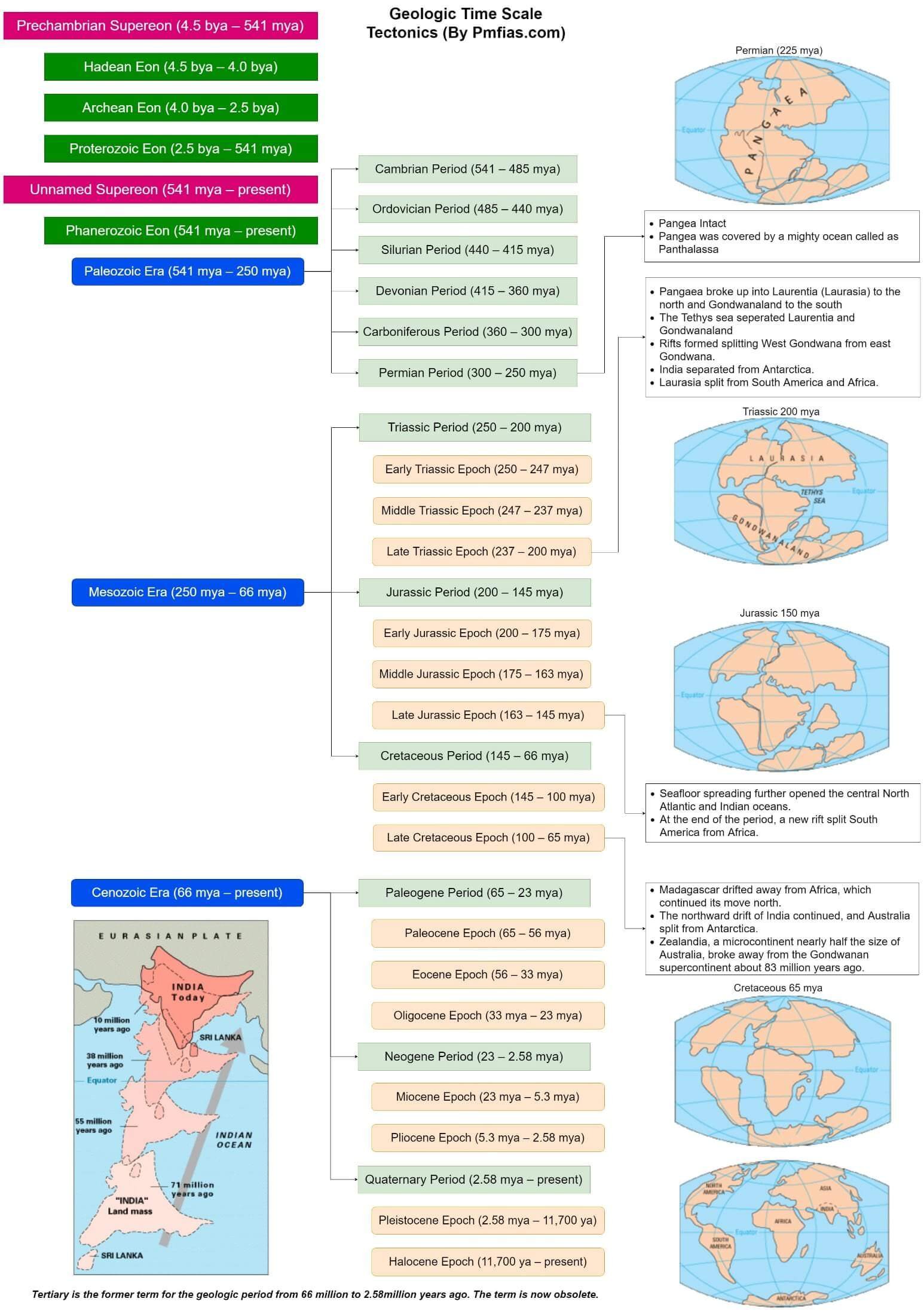
Plate Tectonics – Breakup of Pangea
- Alfred Wegener suggested continental Drift Theory in the 1920’s.
- According to Continental Drift Theory there existed one big landmass which he called Pangaea which was covered by one big ocean called Panthalassa .
- A sea called Tethys divided the Pangaea into two huge landmasses: Laurentia (Laurasia) to the north and Gondwanaland to the south of Tethys.
- Drift started around 200 million years ago (Mesozoic Era, Triassic Period, Late Triassic Epoch), and the continents began to break up and drift away from one another.
Watch the video for quick and better understanding
Forces behind the drifting of continents, according to Wegener
- According to Wegener, the drift was in two directions:
- equator wards due to the interaction of forces of gravity, pole-fleeing force (due to centrifugal force caused by earth’s rotation) and buoyancy ( ship floats in water due to buoyant force offered by water ), and
- westwards due to tidal currents because of the earth’s motion (earth rotates from west to east, so tidal currents act from east to west, according to Wegener).
- Wegener suggested that tidal force (gravitational pull of the moon and to a lesser extent, the sun) also played a major role.
- The polar-fleeing force relates to the rotation of the earth. Earth is not a perfect sphere; it has a bulge at the equator. This bulge is due to the rotation of the earth (greater centrifugal force at the equator).
- Centrifugal force increases as we move from poles towards the equator. This increase in centrifugal force has led to pole fleeing, according to Wegener.
- Tidal force is due to the attraction of the moon and the sun that develops tides in oceanic waters (tides explained in detail in oceanography).
- According to Wegener, these forces would become effective when applied over many million years, and the drift is continuing.
Evidence in support of Continental Drift
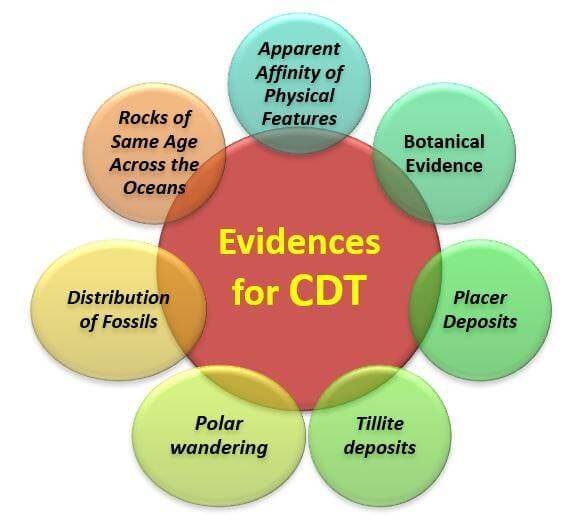
Evidence for CDT
Apparent Affinity of Physical Features
- The bulge of Brazil (South America) seems to fit into the Gulf of Guinea (Africa).
- Greenland seems to fit in well with Ellesmere and Baffin islands of Canada.
- The west coast of India, Madagascar and Africa seem to have been joined.
- North and South America on one side and Africa and Europe on the other fit along the mid-Atlantic ridge.
- The Caledonian and Hercynian mountains of Europe and the Appalachians of USA seem to be one continuous series.
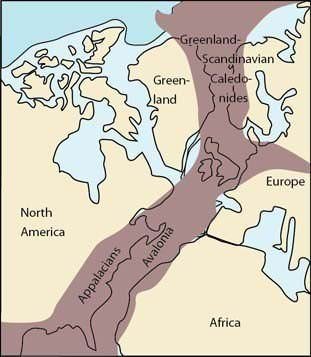
Continuous Very Old Fold Mountain Chain
- Coastlines are a temporary feature and are liable to change.
- Several other combinations of fitting in of unrelated landforms could be attempted.
- Continental Drift Theory shifts India’s position too much to the south, distorting its relationship with the Mediterranean Sea and the Alps.
- The mountains do not always exhibit geological affinity.
Causes of Drift
- The gravity of the earth, the buoyancy of the seas and the tidal currents were given as the main factors causing the drift, by Wegener.
- This is illogical because for these factors to be able to cause a drift of such a magnitude, they will have to be millions of times stronger.
Polar wandering (Shifting of Poles)
- The position of the poles constantly drifted (due to plate tectonics).
- Poles may have shifted, not necessarily the continents.
Botanical Evidence
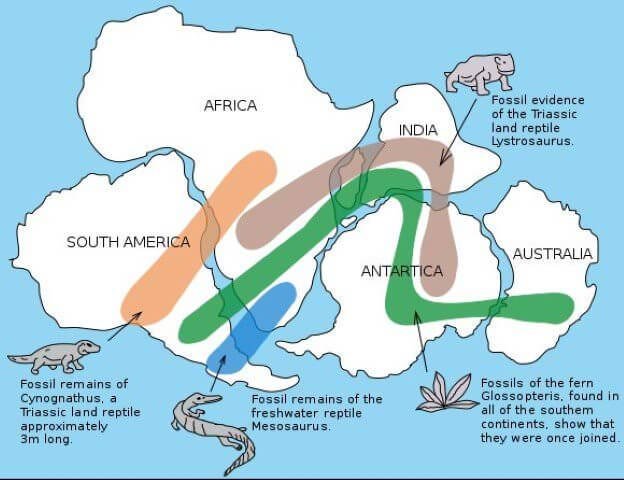
Distribution of Fossils across the Gondwanaland
- Presence of glossopteris vegetation in Carboniferous rocks of India, Australia, South Africa, Falkland Islands (Overseas territory of UK), Antarctica , etc. (all split from the same landmass called Gondwana) can be explained from the fact that parts were linked in the past.
- Similar vegetation is found in unrelated parts of the world like Afghanistan, Iran and Siberia.
Distribution of Fossils
- The observations that Lemurs occur in India, Madagascar and Africa led some to consider a contiguous landmass “Lemuria” linking these three landmasses.
- Mesosaurus was a small reptile adapted to shallow brackish water. The skeletons of these are found only in South Africa and Brazil. The two localities presently are 4,800 km apart with an ocean in between them.
Rocks of Same Age Across the Oceans
- The belt of ancient rocks of 2,000 million years from Brazil coast matches with those from western Africa.
- Rocks of the same age and similar characteristics are found in other parts of the world too.
Tillite deposits
- Tillite deposits are sedimentary rocks formed out of deposits of glaciers .
- The Gondwana system of sediments are found in India, Africa, Falkland Island, Madagascar, Antarctica and Australia (all were previously part of Gondwana).
- Overall resemblance demonstrates that these landmasses had remarkably similar histories.
Placer Deposits
- Rich placer deposits of gold are found on the Ghana coast (West Africa) but the source (gold-bearing veins) are in Brazil, and it is obvious that the gold deposits of Ghana are derived from the Brazil plateau when the two continents lay side by side.
Drawbacks of Continental Drift Theory
- Wegener failed to explain why the drift began only in Mesozoic era and not before.
- The theory doesn’t consider oceans.
- Proofs heavily depend on assumptions that are generalistic.
- Forces like buoyancy, tidal currents and gravity are too weak to be able to move continents.
- Modern theories (Plate Tectonics) accept the existence of Pangaea and related landmasses but give a very different explanation to the causes of drift.
Though scientifically unsound on various grounds, Wegener’s theory is a significant milestone in the study of tectonics, and it laid a strong foundation for future the theories like seafloor spreading and plate tectonics.
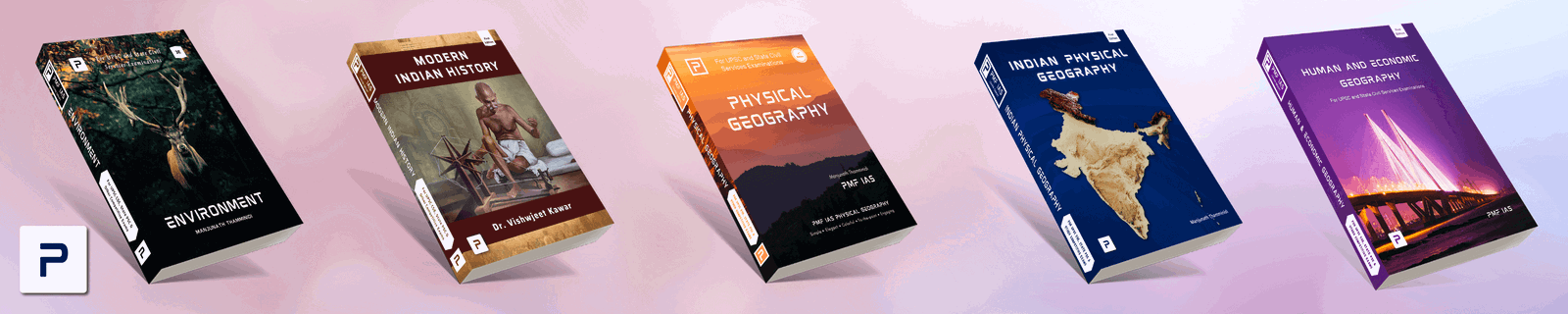
Newsletter Updates
Assured Discounts on our New Products!
Related Posts
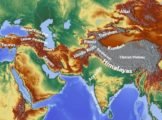
Major Mountain Ranges of the World, Highest Mountain Peaks
- December 29, 2019
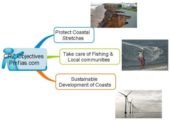
Coastal Regulation Zone – CRZ
- September 26, 2019
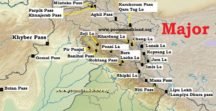
Major Mountain Passes in India & Himalayas
- July 31, 2018
- 11 Comments
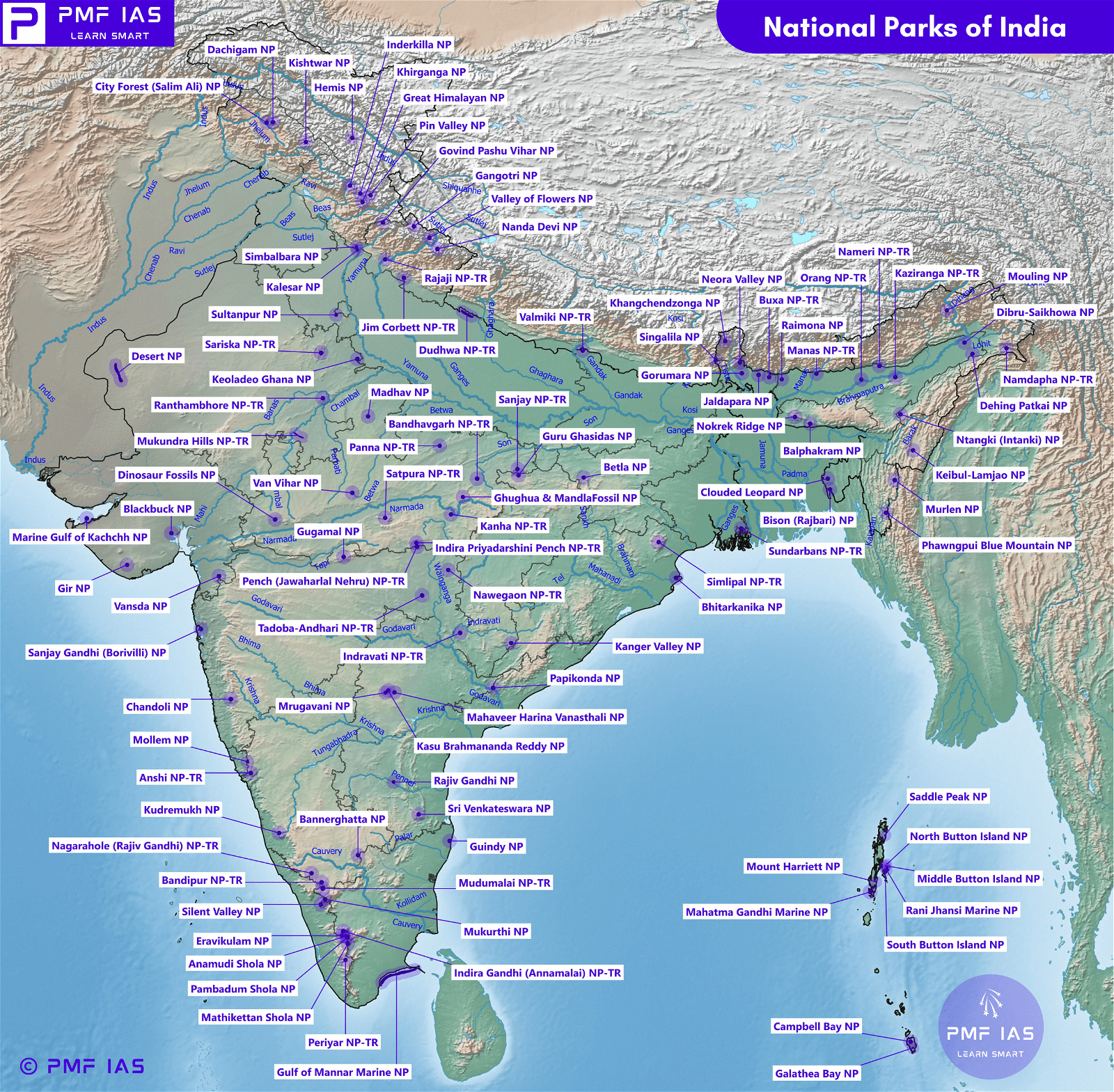
List of National Parks of India (106 National Parks in India in 2022)
- May 22, 2022
13 Comments
Bought and thanks a ton. Really useful for revision.
I want to purchase your notes Geo+Envt+Bio but please let me know should i substitute with standard books or else Your notes + internet source for uncovered topics is enough. what woud you suggest?
please do let me know
Thank You Ramya
My notes + current affairs should be more than enough. You won’t get time to do more than this.
Ok sir purchased yesterday and took print. Thank you very very much. Its really worth. I would suggest every student to go for this notes.
This notes are helful to you Or not
This is helpful to me
Thankyou so much sir… It’s really worthy..
sir actually alfred wegner had proposed continental drift theory in 1912 but what u have mentioned 1922 . that i could not understand pls reply and clarify thanking u sir
Wehner’s theory is of 1912 as mentioned in NCERT class 11. Your source are really helpful however please update such things so that while revision or quick reacap we aspirants don’t learn wrong data. Thank you
Wegner pronounced his concept on continental drift in the year 1912 but it came into light till 1922 when he elaborated his concept in his book..
This is really helpful thanks for the research.
Alfred Wegenar` s theory is in 1912 Please don`n misguide students and you have resposibility to provide correct information beacuse you named as pmf…………whatever Please consider and i really feeling bad while writing this comment because it wasted my most valuable time I think this would be ever critic comment about your content
Leave a Reply Cancel Reply
Your email address will not be published. Required fields are marked *
Name *
Email *
Add Comment *
Post Comment
Trending now

Never miss an important update!
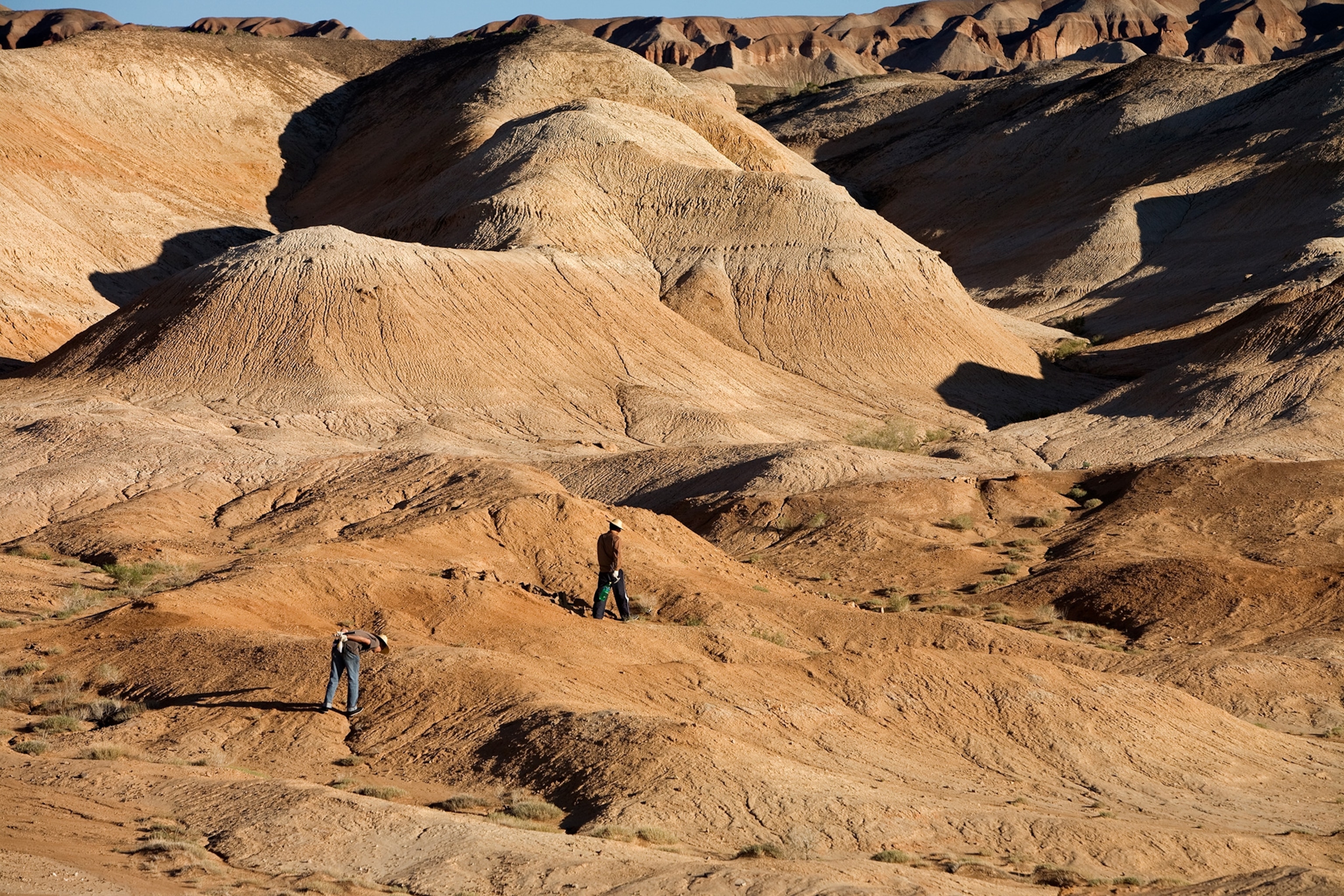
Scientists scour the Junggar Basin in northern China. Rocks across the country record dramatic climate change between 165 and 155 million years ago. Older rocks are rich in coal that formed in cool and wet conditions, while younger red sandstones formed in hot and dry climes. Researchers now suggest this alteration has a curious cause: a change in the region's geographic location linked to a phenomenon called true polar wander.
Earth's odd rotation may solve an ancient climate mystery
A geologic change might have plunged lush landscapes into arid zones, killing off an array of creatures—and it might happen again one day.
At first, it seems like a case of extinction by climate change: More than 160 million years ago, during the Jurassic period, a fanciful menagerie crept, swam, and flew through the cool, damp forests of what is now northeastern China. Then, almost in a geologic instant, the air grew warmer and the land dried out. As the water disappeared, so too did the life. And yet, researchers have struggled to pin down a climate-related culprit behind this ecological collapse.
Now, a study published in the journal Geology suggests that it wasn’t the climate that changed, but the geographic location of the landscape . Paleomagnetic signatures in the area’s rocks indicate that sometime between 174 and 157 million years ago, the whole region shifted southward by a startling 25 degrees, plunging once lush landscapes into zones of desiccating heat.
The ancient rocky lurch was part of a phenomenon known as true polar wander, in which the topmost layers of the planet, likely all the way down to the liquid outer core, rotate significantly even as Earth continues its daily turn around its usual spin axis.
In the Jurassic, the surface and mantle made this twist around an imaginary line through the crook in Africa’s west coast known as the Bight of Benin . The change would have been massive: If a similar shift were to happen today, a flag planted in Dallas, Texas, would end up where Northern Manitoba, Canada, currently sits. On the other side of the world, the continent of Asia would soar southward.
Earth has likely experienced smaller amounts of true polar wander throughout its past, and some scientists think it continues today.
“We’re experiencing true polar wander as we speak,” says Dennis Kent, a paleomagnetist at both Rutgers and Columbia University who wasn’t part of the new study team.
Nat Geo Kids Back-To-School Exclusive Offer
Get up to 4 bonus issues!
To be clear, these more recent forays are not the source of modern-day climate change, which is driven by humans’ relentless release of greenhouse gasses into the atmosphere . In addition, the magnitude of this Jurassic shift—and whether true polar wander is even a real phenomenon—remain under debate.
“It’s a reasonable area of discussion,” says Christopher Scotese , director of the PALEOMAP Project. “But it’s more controversial than people give it credit for.”
Studying Earth’s past and present geologic wanderings may not only help resolve the controversy, but also improve our understanding of the planet’s complex machinations.
“It’s so important that there’s still fundamental science being done,” says Lydian Boschman , a geologist at Eidgenössische Technische Hochschule (ETH) Zürich who was not a study team member. “If we don’t understand the foundations, then there’s nothing we can do on top of that.”
Twisty-turny past
While deep geologic gyrations can have drastic impacts on Earth’s surface, the planet’s magnetic field remains largely unchanged by such events, since it is generated by the churn of molten iron and nickel in our planet’s outer core, some 1,800 miles below the surface. Researchers can therefore turn to iron-rich minerals attuned to magnetic fields to untangle the planet’s past twists and turns. As sediments collect and solidify or lava cools to stone, these minerals align themselves with the global magnetic field like compass needles, recording a snapshot of a region’s location on our planet at a given period in the past.
But not all rocks are perfect stenographers. As sediments are turned to rock, compression can tweak the magnetic signature and impact their inferred planetary position. By removing this sedimentary confusion and looking only at volcanic rocks, Kent and the late Edward Irving , who worked at the Geological Survey of Canada, found the signatures of a monster jump during the Jurassic period . Their results, published in 2010, suggested that Earth’s surface shifted some 30 degrees between 160 and 145 million years ago.
Subsequent studies started to fill in the gaps in the record, and increasingly it seemed that the entire world was in on the Jurassic mega-shift, with evidence found in modern-day Africa , North America , South America, and the Middle East . But one place appeared to largely stay put: the Eastern Asian Blocks, a zone that includes most of Mongolia, China, North Korea, and South Korea.
“It hardly moved in terms of latitude during that entire period,” says study coauthor Joseph Meert , a paleomagnetist at the University of Florida. “That didn’t seem to really jive well with aridification.”
Part of the challenge was that studies documenting the region’s position with paleomagnetic analyses didn’t sample from a large enough swath of time, Meert explains. While volcanic rocks faithfully record magnetic north, this pole has a tendency to roam, so researchers must average their analyses with data covering several thousands of years to account for these wanderings. ( Magnetic north just changed, and here's how we’re trying to keep up. )
The region itself was also frequently excluded in discussions of global change because of its complex history, Kent adds. While the path of other landmasses can be traced back to the supercontinent Pangea, which broke up roughly 180 million years ago , East Asia’s route remains unclear.
You May Also Like
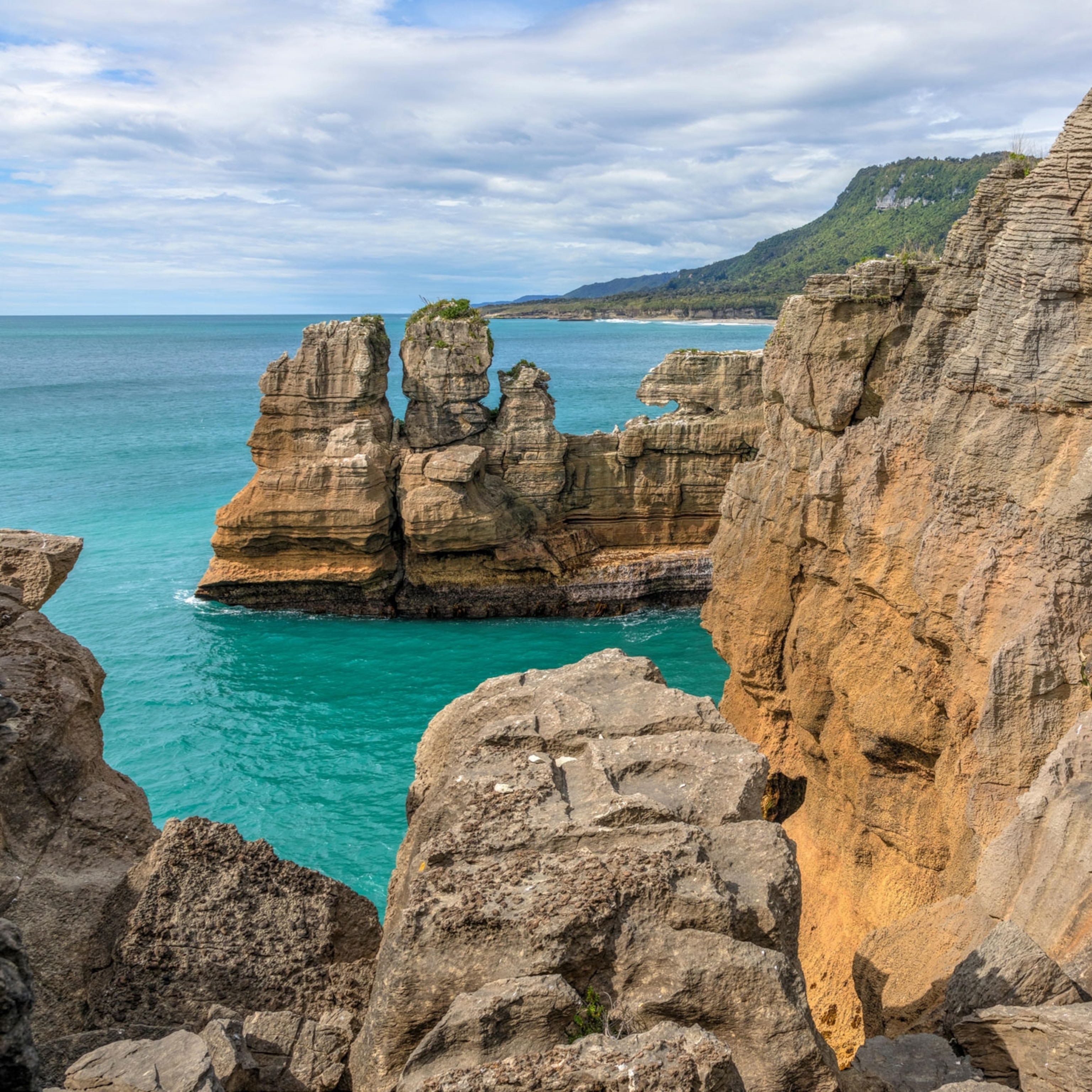
The lost continent of Zealandia has been mapped for the first time
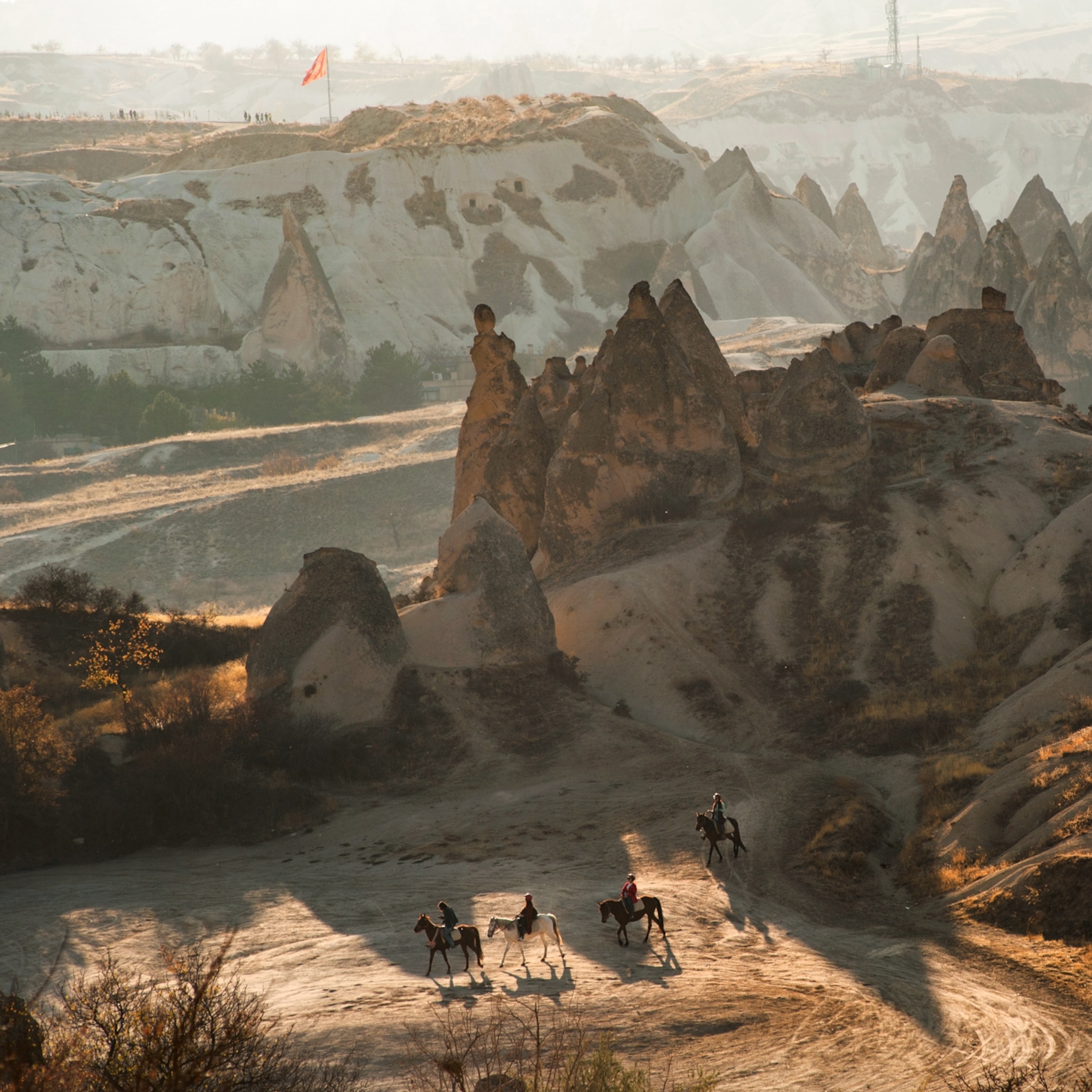
The surprising source of Turkey's volcanoes lies more than 1,000 miles away
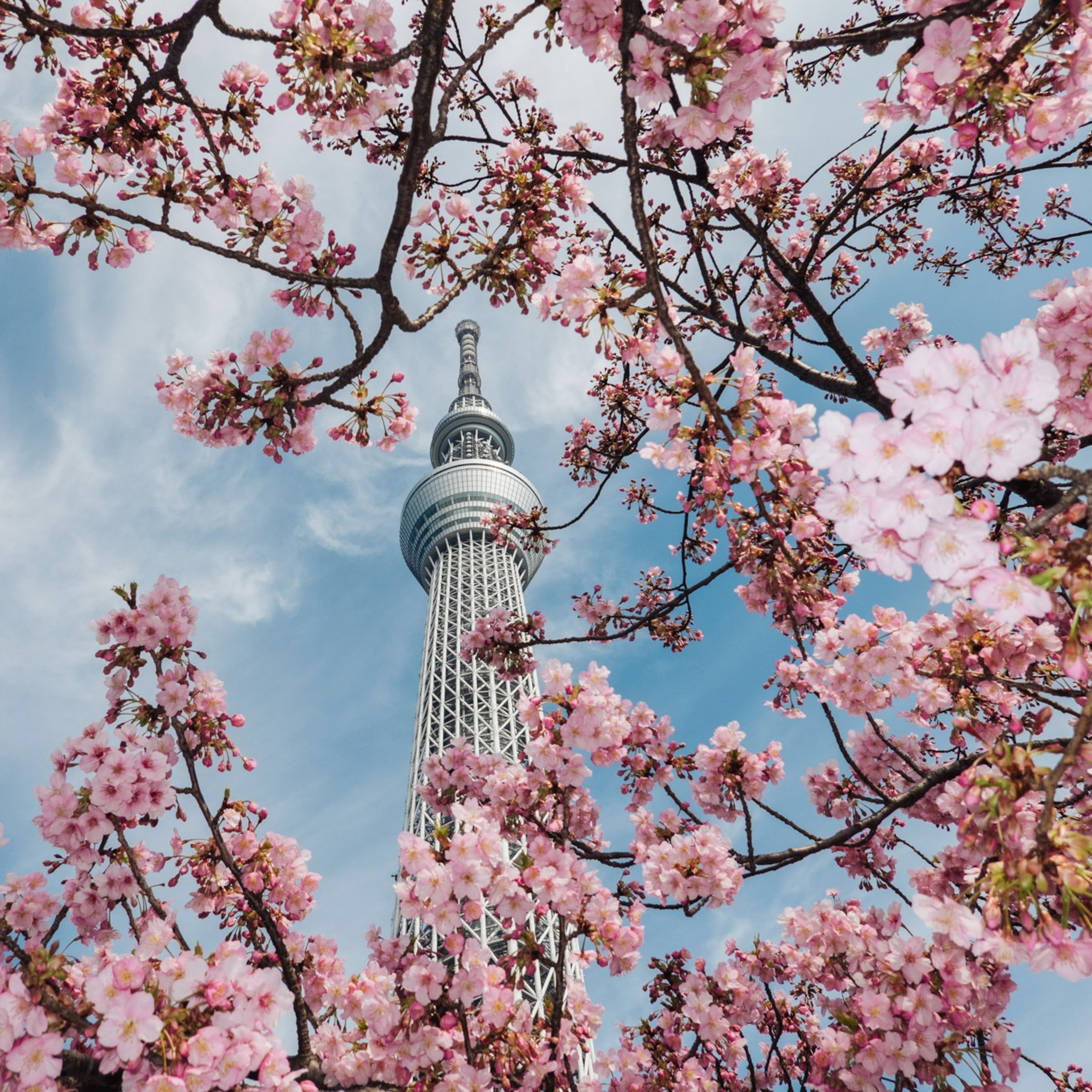
Japan spent decades making itself earthquake resilient. Here's how.
“They were doing their own dance out there,” Kent says.
The monster shift
In the summer of 2015 and spring of 2018, the latest team set out in search of a more robust paleomagnetic record to untangle the geologic movements of East Asia, says lead study author Zhiyu Yi of the Chinese Academy of Geological Sciences in Beijing.
Rocks across China tell very different stories, as portrayed by their starkly different hues. The early to middle Jurassic deposits are dark and rich with coal, hinting at a humid ancient landscape chock full of plants. By contrast, the late Jurassic formations hosted rusty red deposits laid down in drier conditions. ( Learn about the bizarre fossil finds that revealed Asia’s oldest known forest .)
The team sampled volcanic rocks interwoven into these contrasting formations at a total of 57 sites. In 2017, their analyses confirmed past work that showed the younger red rocks were laid down at low latitudes, where hot and dry conditions likely prevailed, Yi says. But the moment of truth came in the summer of the following year, when they analyzed the older samples and discovered that they formed at surprisingly high latitudes.
“At that moment, I knew what these data mean to us—we finally found the [true polar wander] signals,” Yi writes via email.
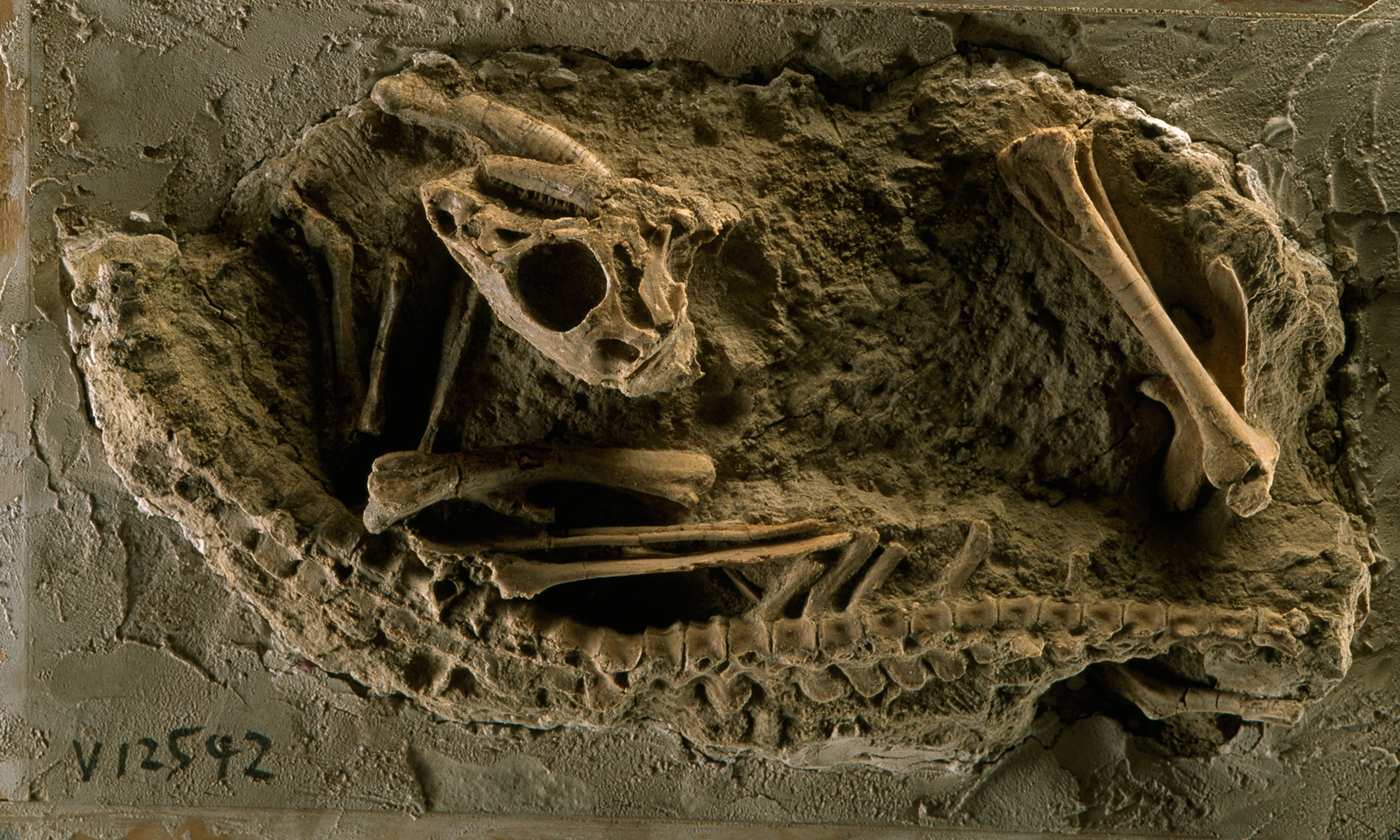
True polar wander may be behind the demise of northern China's array of life known as the Yanliao Biota. This die-off set the stage for a new jumble of creatures to arise known as the Jehol Biota, which includes the fossil Jeholosaurus shown here.
Meert admits that he was a little skeptical of the massive shift at the start, but the new findings have him convinced: “We were saying, Yes, yes, this is it,” he says, recalling the time he sat down to dinner with Yi in Beijing to review the data. “The sense of motion and everything just seemed to fit neatly together. So we had a beer and toasted and said, Let’s do this.”
The results suggest that the Jurassic surface rotated by at least 6.7 inches each year, which led to the slow drying of the East Asian landscape that likely killed off many of the region’s ancient plants and animals known as the Yanliao Biota. Past studies hint that another smaller wander around 130 million years ago returned East Asia to temperate climes, setting the stage for the rise of a burst of life known as the Jehol Biota . These exceptionally preserved fossils have yielded many startling finds, including the discovery of the first known feathered dinosaur that was not directly related to birds. ( Read about the dinosaurs that didn’t die. )
Spinning futures
“The beauty is that it is very simple,” says Giovanni Muttoni , a paleomagnetist at the University of Milan, Italy, who was not involved in this work but has extensively studied the big Jurassic wander. The motion and magnitude line up with past work, he notes, and they connect mysterious changes in climate with this planetary twist.
However, Scotese isn’t convinced that true polar wander occurred at all during the last 200 million years, arguing that the effects could be explained by the movement of tectonic plates. During the Jurassic period, he says, Asia and North America inched along as if they were on a seesaw that pivoted around Europe. While North America moved northwest, Asia shifted southeast.
“There’s a huge amount of noise in the paleomagnetic database, and often paleomagnetists do all sorts of contortions to try to minimize the noise or correct things that they think are errors,” he says. “I just disagree with that philosophy. I feel that’s biasing the database.”
Others stand firm with the data pointing to true polar wander and the monster shift in the Jurassic.
“They are real,” Muttoni says. “They are recorded in the rocks.”
If so, many questions linger. For one, it’s unclear precisely what drives such a large shift, an event that must involve some significant redistribution of our planet’s mass. Perhaps the birth of subduction zones —regions where one tectonic plate drives under another—drives the wander, Boschman says. Or, it could be due to slabs that have already subducted breaking apart, which would then send pieces of crust sinking through the mantle, upsetting the planetary balance, Kent adds. For now, unraveling the many geological unknowns is all part of the intrigue.
“We’ve got a nice expanding envelope of knowledge, and you try to be at the meniscus where it’s happening,” Kent says. “But in the meantime, you may be out there on a ride.”
Related Topics
- CLIMATOLOGY
- EXTINCT SPECIES
- PLATE TECTONICS
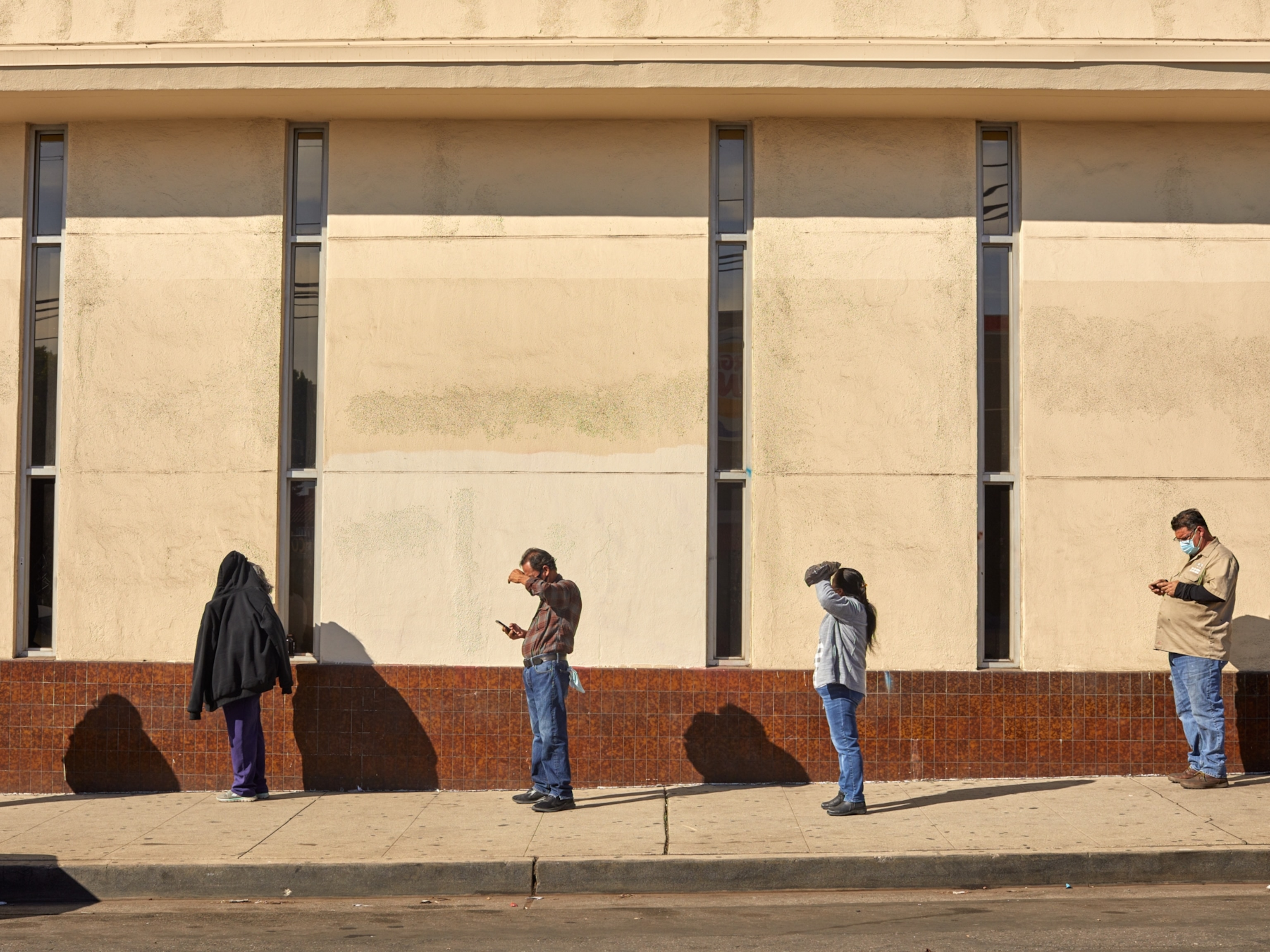
Extreme heat is the future. Here are 10 practical ways to manage it.
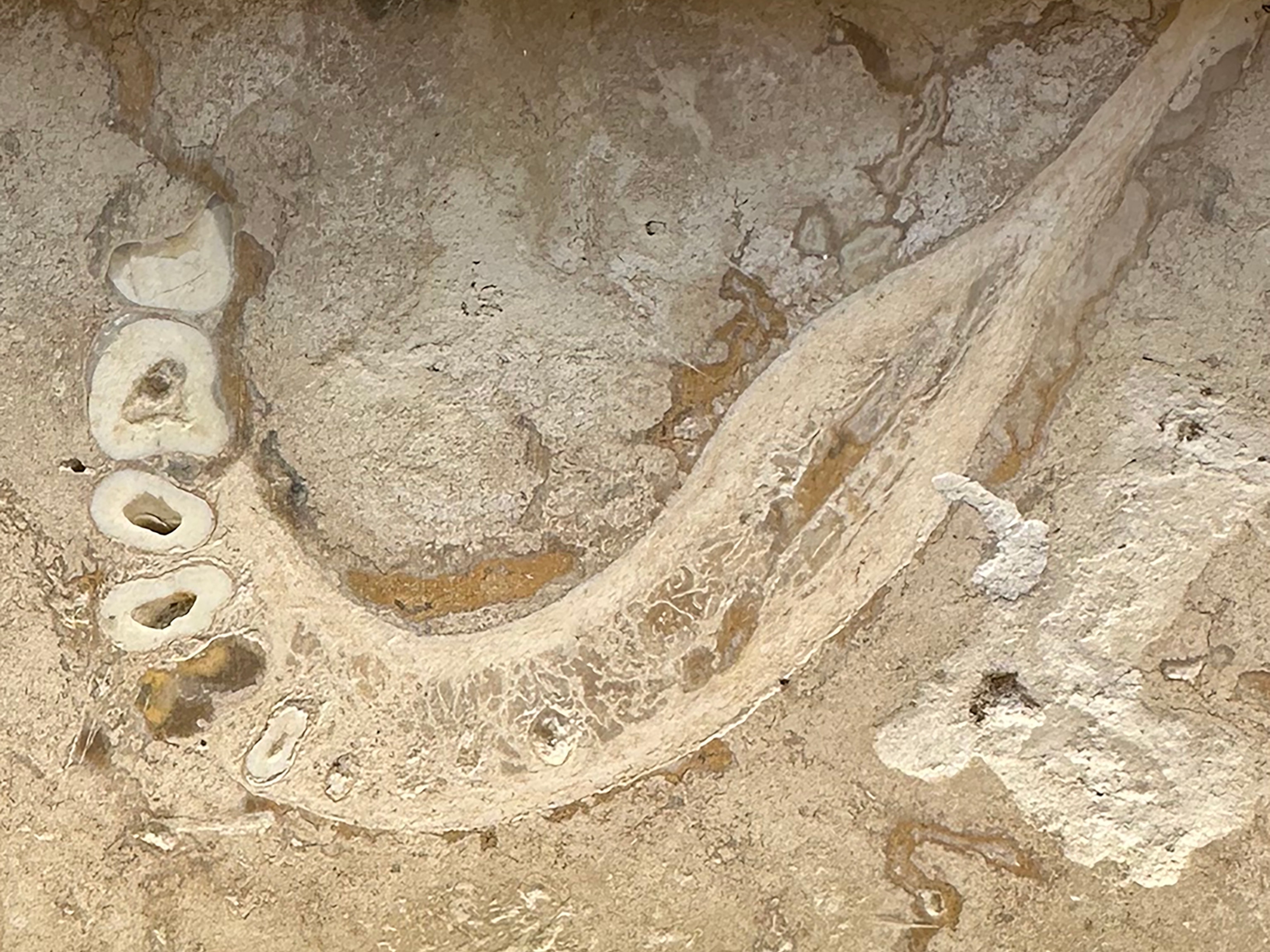
Your tile floor may contain human fossils
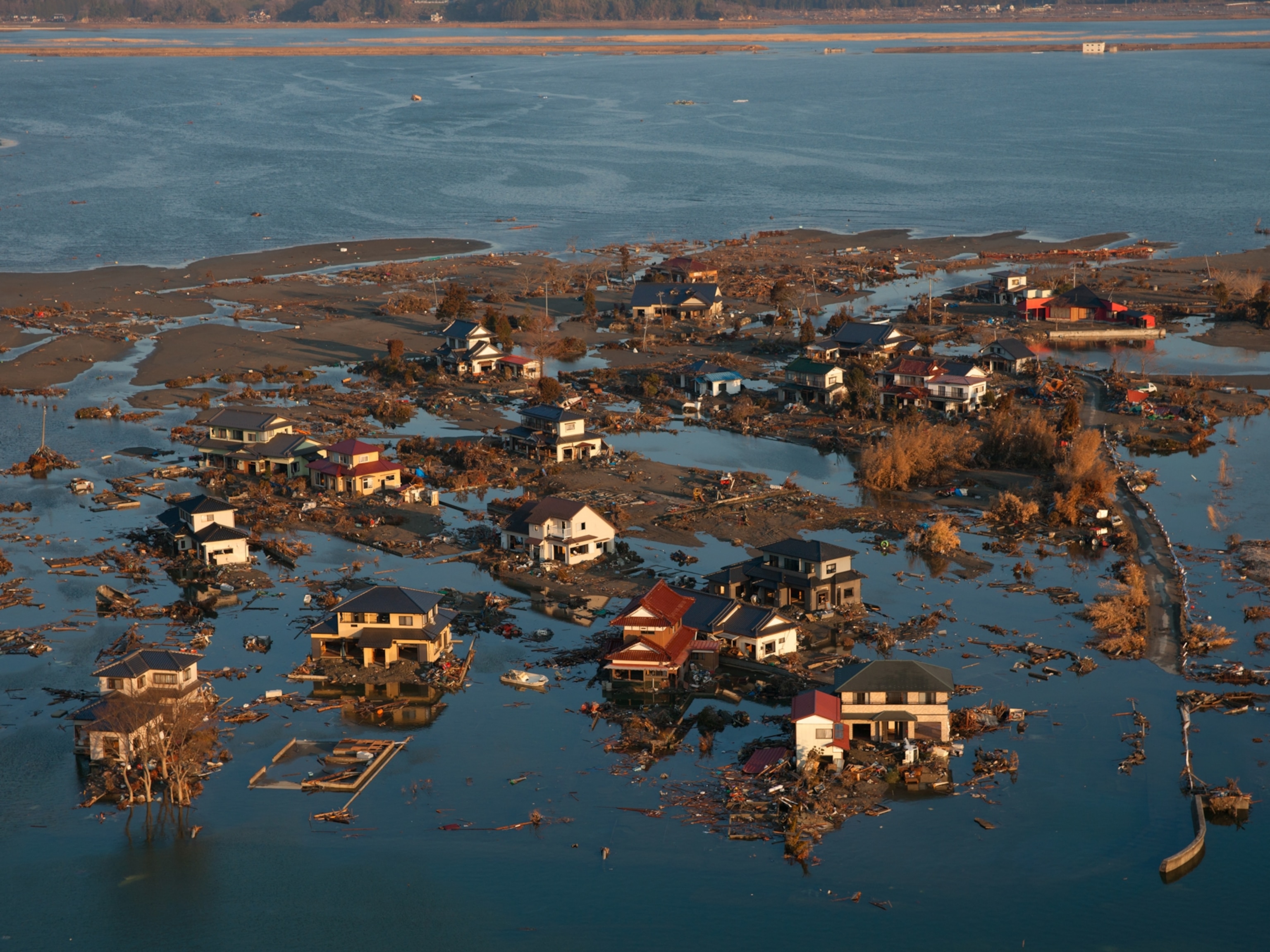
Japan's 2011 megaquake left a scar at the bottom of the sea. Scientists finally explored it.
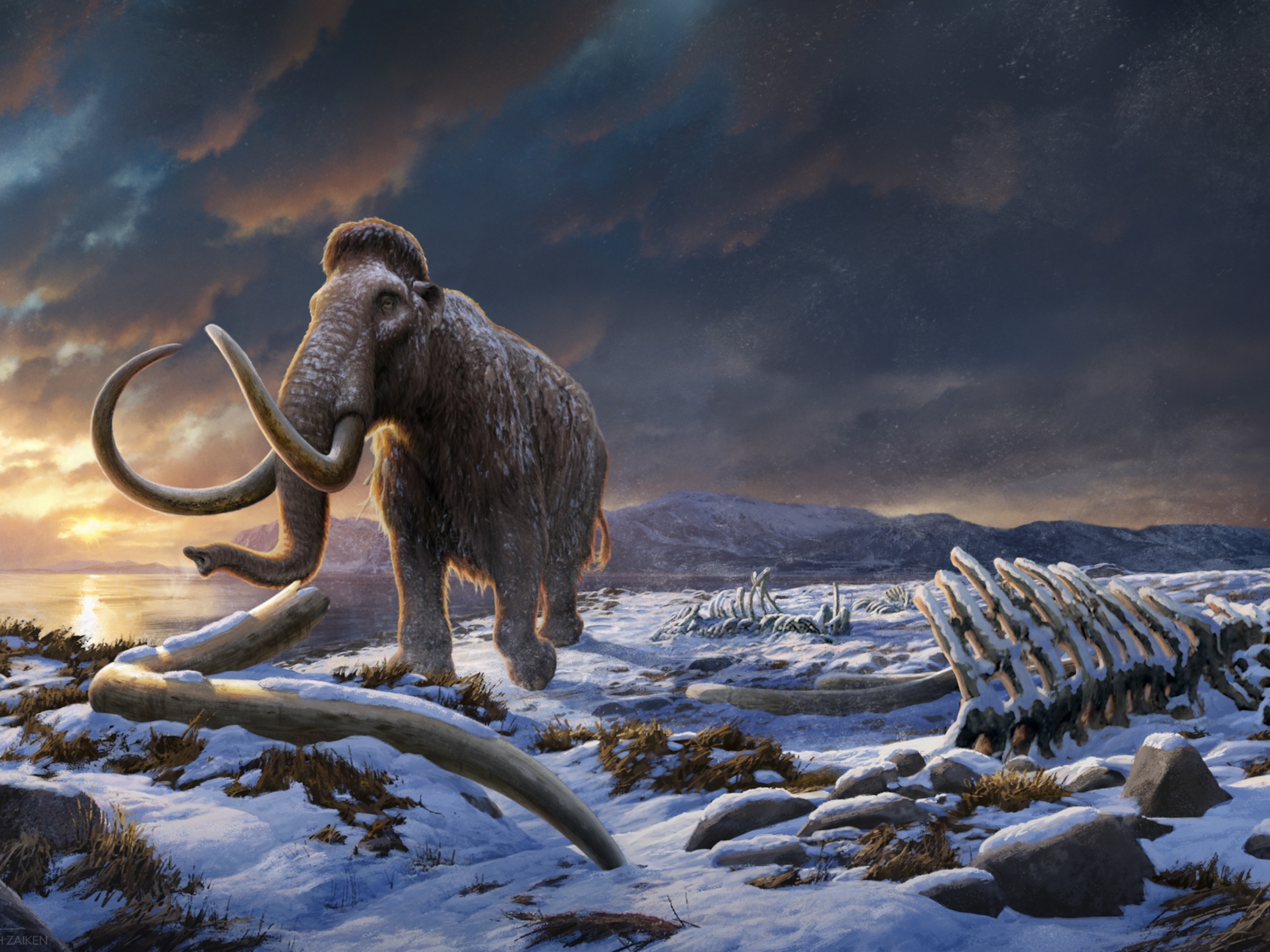
What killed the last woolly mammoths?
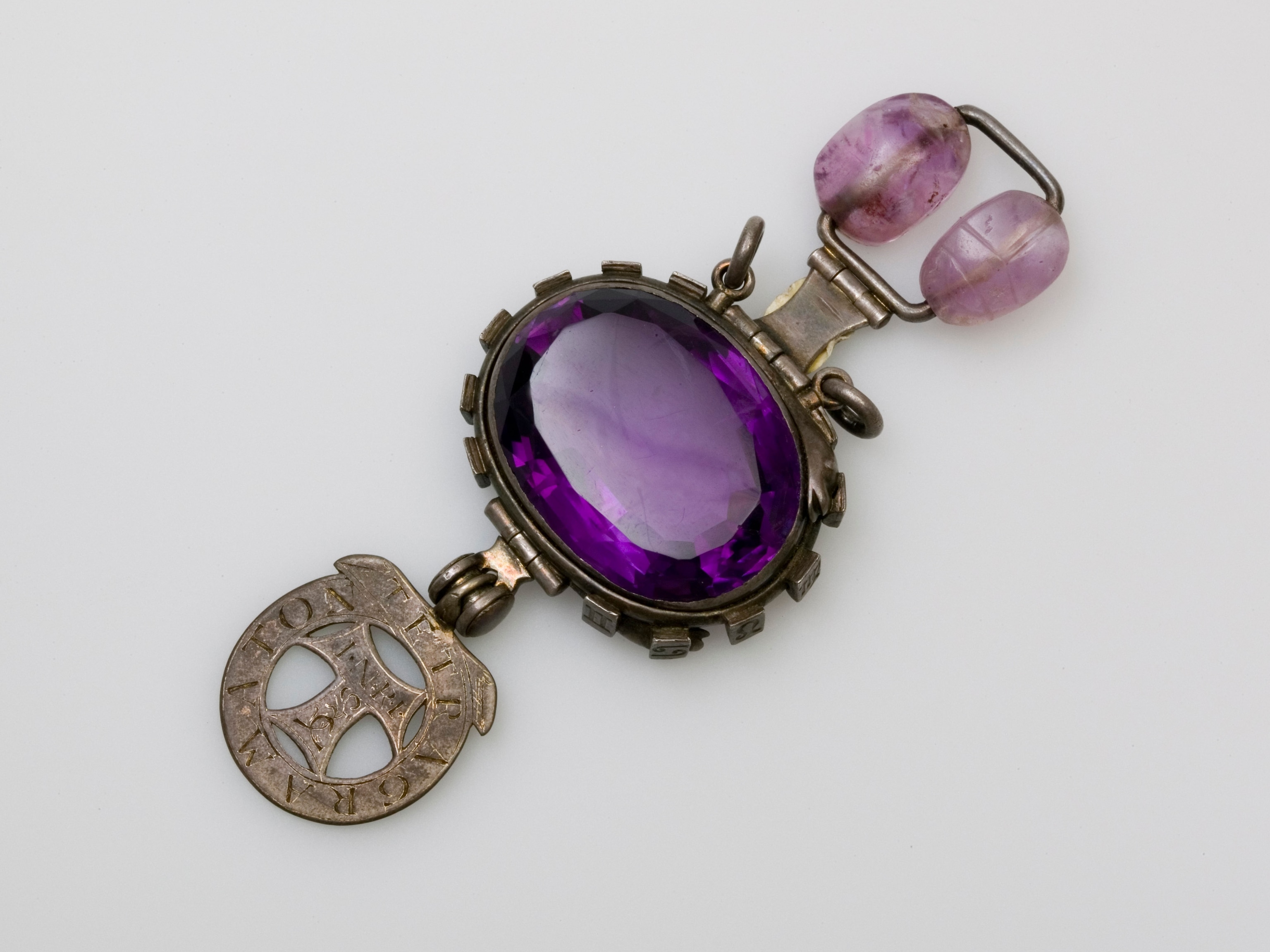
This legendary gemstone is real—but was it actually cursed?
- Interactive Graphic
- Environment
History & Culture
- History & Culture
- The Big Idea
- Paid Content
- Terms of Use
- Privacy Policy
- Your US State Privacy Rights
- Children's Online Privacy Policy
- Interest-Based Ads
- About Nielsen Measurement
- Do Not Sell or Share My Personal Information
- Nat Geo Home
- Attend a Live Event
- Book a Trip
- Inspire Your Kids
- Shop Nat Geo
- Visit the D.C. Museum
- Learn About Our Impact
- Support Our Mission
- Advertise With Us
- Customer Service
- Renew Subscription
- Manage Your Subscription
- Work at Nat Geo
- Sign Up for Our Newsletters
- Contribute to Protect the Planet
Copyright © 1996-2015 National Geographic Society Copyright © 2015-2024 National Geographic Partners, LLC. All rights reserved
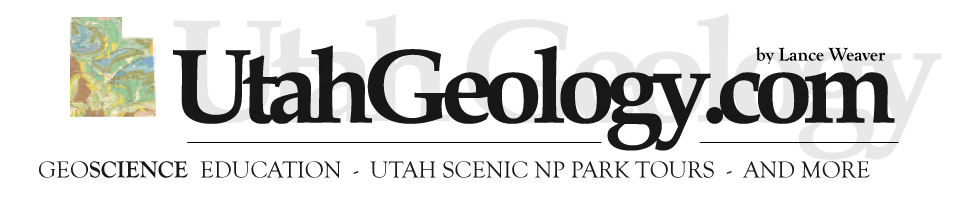
Could the Ice Ages have been True Polar Wandering Events?
This is one of those things that once you see, you can’t un-see.
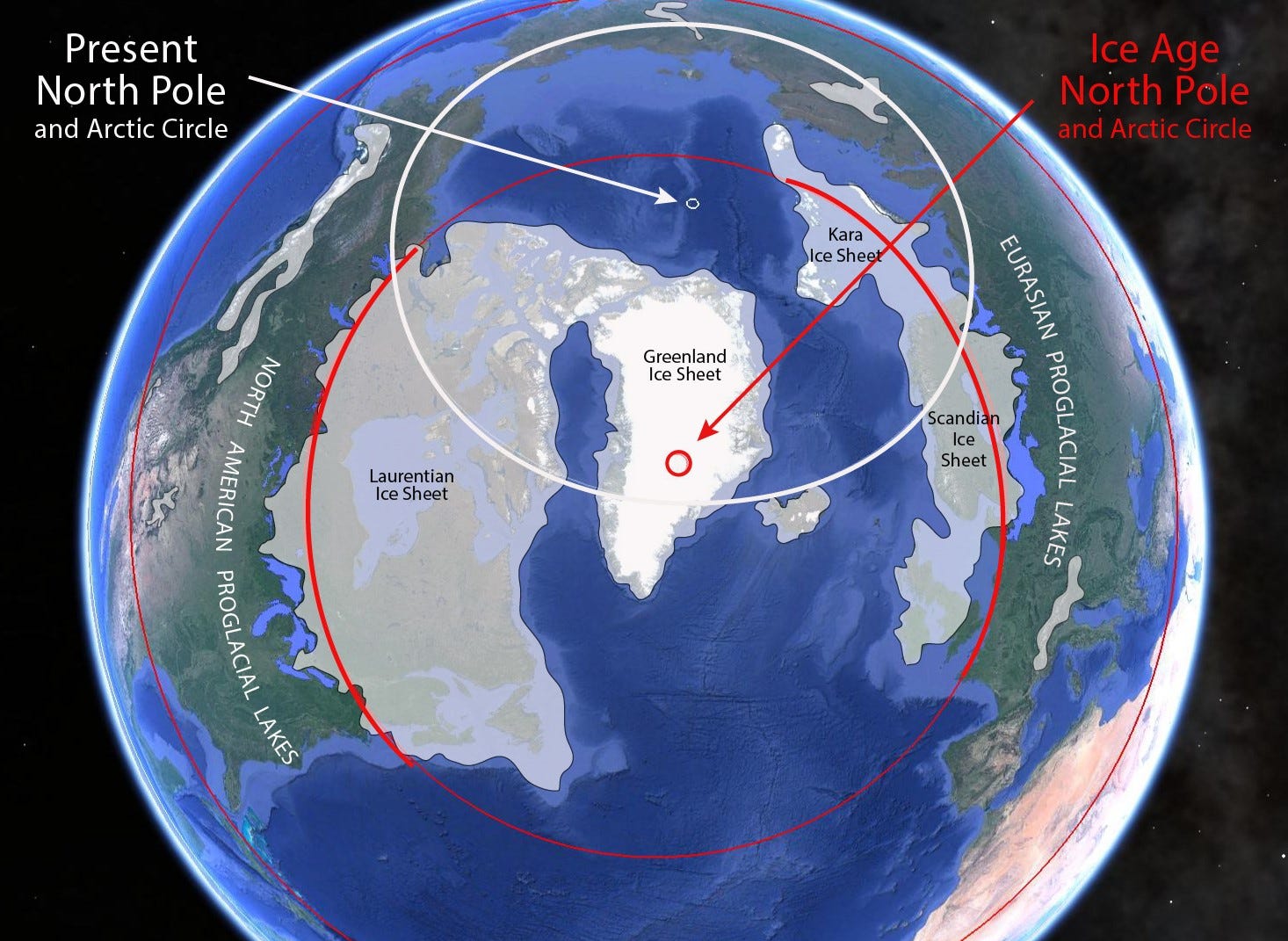
Introduction to the Rogue Theory
It was during my undergraduate or graduate work toward my degree in geology and geophysics that I first noticed that the majority of Northern Russia, Siberia and Northern Alaska were never fully glaciated during the Ice Age. In fact the areas where the last continental ice sheets persisted formed a nearly perfect ‘Arctic circle’ around a pole centered over Greenland.
The more I puzzled over this, the odder it seemed to me that the earth could be cold enough during the ice age for arctic ice to extend to 50 degrees south of the present Arctic Circle and into parts of Illinois and Germany (literally half way to the equator), and yet parts of Alaska and Siberia which are within the present arctic circle were never covered by continental ice sheets or glaciers!
How could this be? For years I’ve searched for a believable answer, always finding the same unconvincing response thrown out. “Siberia & Alaska were an arctic desert, and because of their distance from the sea — storm cells could not carry moisture far enough inland to those areas.” A pretty terrible response in my opinion considering the exact same “arctic desert” conditions would have existed over the 8,000 feet of ice in Central Canada during the Pleistocene, and even currently exist in the center of Antarctica and yet there’s still upwards of 10,000 feet of ice there today! If there’s one thing Antarctica teaches us, its that ice sheets still form in ‘Arctic deserts’ or regions where very little snow falls. In the long run continental ice sheets have a lot less to do with annual snowfall totals, and a lot more to do with temperatures being low enough to limit melting. More sophisticated answers involving anticyclones, Hadley cells in the jet stream driven by particular ocean currents and intercontinental rain shadows have been offered but are all equally implausible when examined closely.
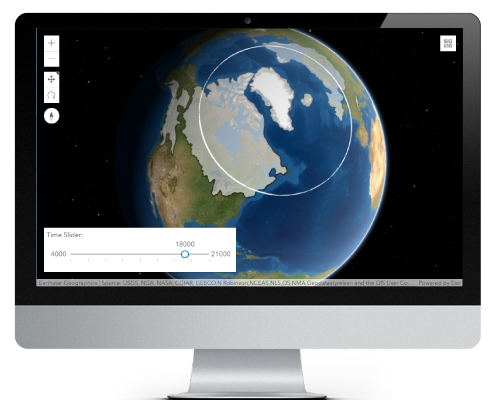
Ice Cover Visualization . Click on the link to see our tool which visualizes the extents of the Pleistocene polar ice sheets in a 3D interactive ice age map viewer . Slide the time slider to see the ice sheet extents though time as well as the theorized track of the north pole. Toggle on & off current & ancient latitude lines as well as an Antarctica overlay.
Scrutinizing the predominate explanation
Central Antarctica really is a frozen desert. The Amundsen-Scott South Pole Station , located in the middle of Antarctica typically records only about 0.5 – 3.1 inches of snow (water equivalent) per year. Most of its snow accumulation there is often blown in from the coastal regions where snow fall can be as high as 15–25 inches a year. (Or it falls as frost-like ice crystals instead of snow) The continent as a whole averages only 6 inches of snow a year, and yet still has managed to accumulate 1 to 3 miles of ice over the last 14 million years (5,000-13,000 feet of ice). Compare that to Fairbanks in the center of Alaska which averages around 45 inches of snow a year but zero glacial accumulation — and you can see how its not impressive snowfall totals that form continental glaciers but consistently cold summer temperatures low enough to facilitate less snow melting than gains.
As anyone whose spent much time in alpine environments knows. Its the night time temperatures that dictate when the snow and ice is about to begin its rapid summer melt. Glacial science is complex, but as a general rule, if you want to grow a glacier, all you need is ANY snow and temperatures which are consistently BELOW freezing in the accumulation zone. Places like Prudhoe Bay or Fairbanks Alaska with summer night time temperatures of 40°F to 50°F are simply not cold enough to grow or maintain glaciers, despite their high snowfall totals. On the other hand places like Casey or Esperanza Base in Antarctica, with summer night time temps of 20°F to 30°F, with smaller snowfall totals are.
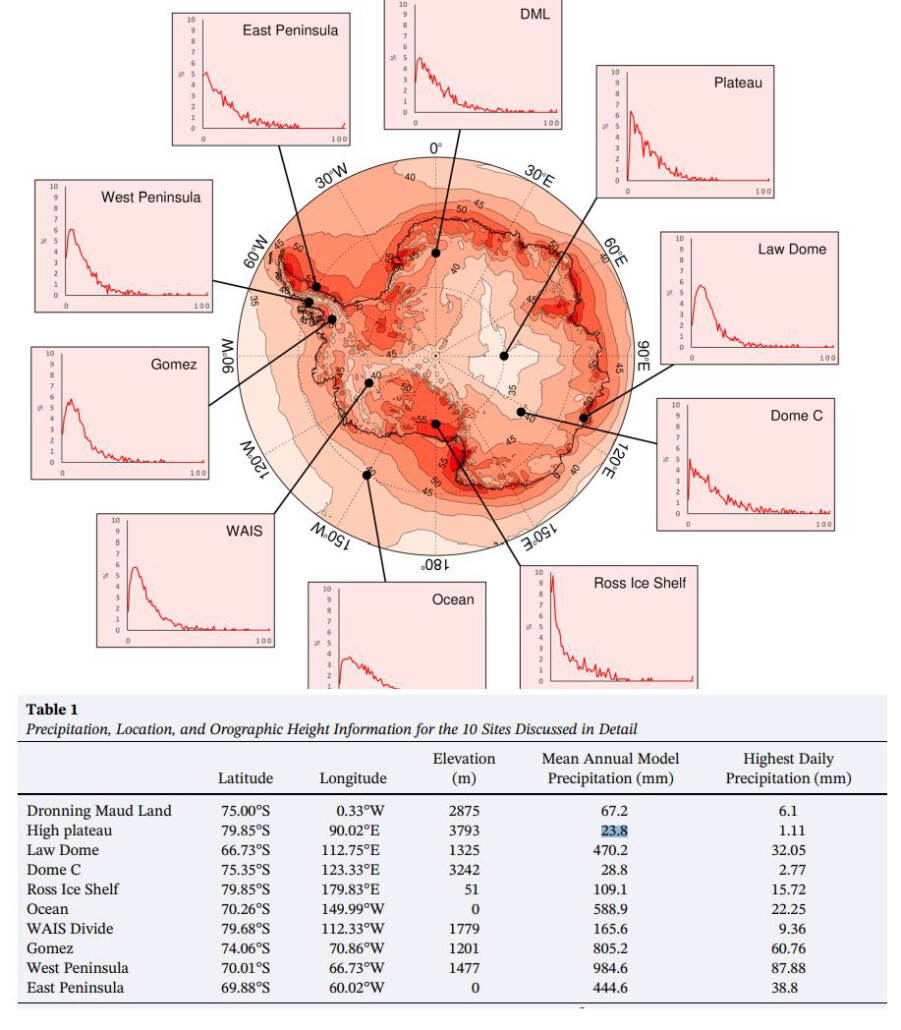
So how is it then that places like Illinois, New York, Denmark and North Germany with current summer night time temperatures up to 60-70°F, and latitudes of 46° (roughly 5,500 miles from the North Pole), were able to accumulate upwards of 7000-10,000 feet of glacial ice during the supposedly frigid Ice Age. And yet places like North Siberia or Northernmost Alaska, with current summer night time temps as low as 40°F and latitudes within the Arctic Circle (2,500 miles from the North Pole) were not? The current “frozen desert” explanation holds less water than the frozen air that many blame it on. Really, its borderline ridiculous. Especially when you consider places like central Alaska or the Kamachatka region of Eastern Russia. These regions fall at the tail end of strong North Pacific weather cells which massive amounts of moisture from the Pacific to these Northern Latitudes. (Just like Greenland, the North Sea & Scandinavia are at the tail end of the Caribbean Jet Stream which does the same in that hemisphere). To suggest that these coastal regions did not have access to enough moisture to build ice sheets is as unlikely and faulty as the argument that ice sheets need large amounts of snow to build in the first place!
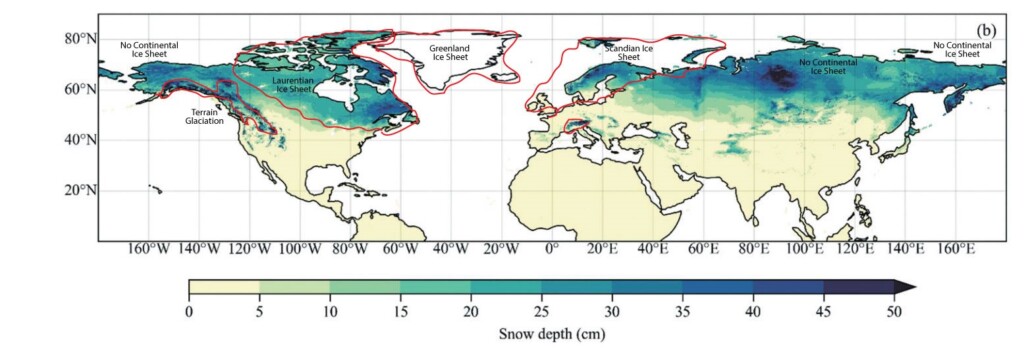
As a great example lambasting both these arguments, look at the annual snow accumulation portrayed from time-lapse satellite imagery of the Northern Hemisphere. The annual snow gains and losses follow latitude almost exactly with the only exceptions being high elevation areas like Greenland & the Rockies. The current winter Arctic desert conditions of Central Siberia make little to no difference in the general trend of annual snow cover. Despite the smaller amounts of snow, high latitude regions like Siberia, Northern Alaska and Arctic Canada are the first to gain snow, and the last to lose it each year (or high elevation regions like Greenland & the Northernmost Rocky Mountains which are cold enough to keep ice all year long).
See the same yearly snow and ice accumulation with sea ice added, and note how it corresponds almost entirely to latitude. Only elevation, as already mentioned, and the Gulf Stream current, which brings warm water from the South Atlantic to the North Sea affects the general latitudinal rule of snow accumulation—making areas of northwest Europe slightly warmer, a trend opposite of what we sea during the ice age.
Glacial geologists sometimes also use processes like the albedo effect , thermohaline oceanic currents and localized microclimates created by ice or large fresh water dumps into constricted oceans to help explain how continental glaciers could have been SO massive and globally lopsided during the Ice Age. As evident in the above snow accumulation maps, every one of these three afore mentioned factors obviously have only little effect on modern day glacial or ice sheet growth. Truly the lack of ice in the North Sea caused by the Gulf Stream is the only real example of a microclimate created by ocean currents which breaks the general latitudinal rule of snowfall and snow/ice persistence. And even its strong effects only change latitudinal snow persistence by around 15 degrees latitude—and even then the effect is restricted primarily to marine ice and coastal environments.
Some have suggested that Greenland’s ice sheet existing at such a southern latitude is another exception to the latitudinal rule of snow persistence, however, I find those who see Greenland as a glacial anomaly, haven’t actually studied the elevation profile of the continent in order to see how the high the mountain ranges which literally surround the continent are (especially the southern tip of the continent which extends out of the arctic circle). These mountains are the obvious explanation for why Greenland has maintained an icecap post-Pleistocene at latitudes farther south than adjacent iso-latitudinal ice sheets. And were the formation of the Greenland ice sheet or its persistence predominately any of the other three reasons (albedo effect, oceanic currents or localized microclimates caused BY the ice) we would SURELY have had a similar Pleistocene ice sheet in the Kamchatka region of Eastern Russia or an existing persistent ice sheet somewhere in Siberia or Eastern Russia since it would have been subject to the same Albedo Effect — and to this day consistently has one of the coldest non-glacial microclimates on earth!
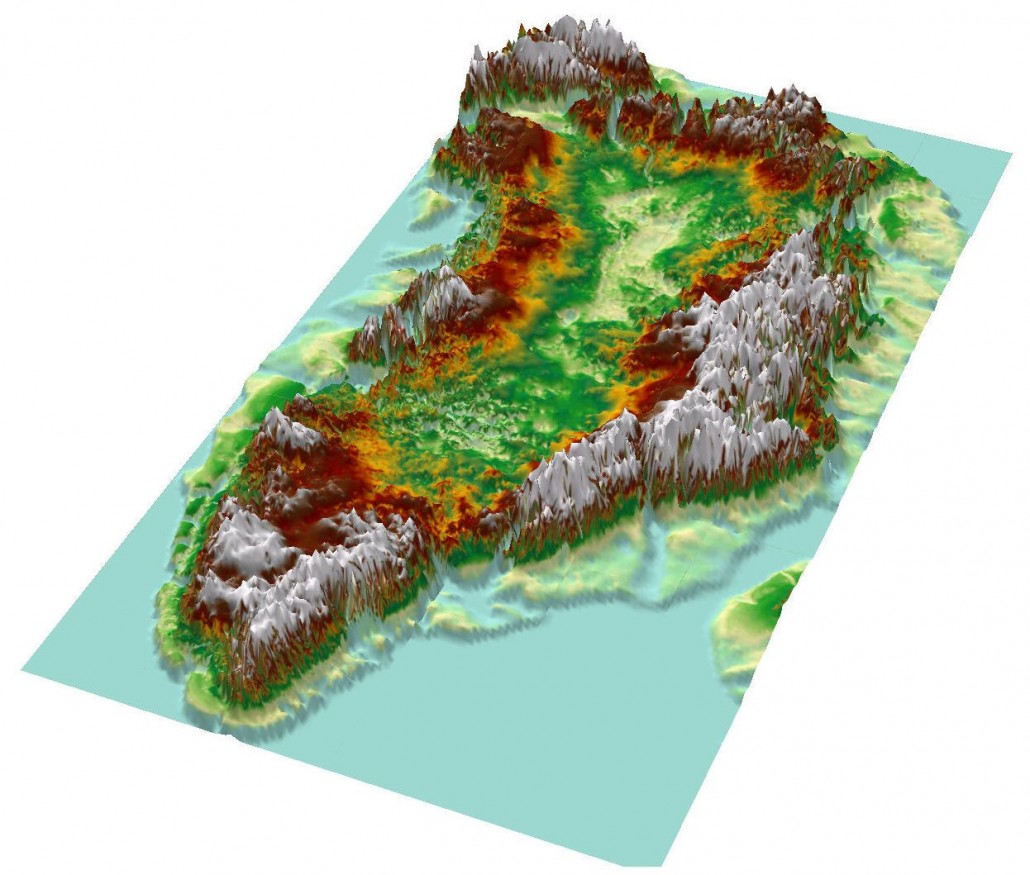
Growth & Melting of Ice Age Ice Sheets
Now let’s compare the above snow accumulation animations to an animation of the glacial ice growth and retreat during the last ice age. Pleistocene snow and ice accumulation and melting followed an entirely different pattern than it currently does. In fact it is striking how obviously the ice sheets seem to point toward a geographic north pole in the region of Greenland.
There’s a lot of misunderstanding among the unstudied general public on exactly where the great northern Ice Sheets were and were not during the ice age. (There is near total agreement among trained glacial geologists). This public misunderstanding is mostly the result of poor illustrations based on imagination instead of science. Among experts the northern Ice Sheets and their terminal moraines have been well mapped & dated, with the features proving past continental glacier’s locations being well understood. In fact, in addition to the typical continental glacier telltale signs such as bedrock scour, eskers, outwash plains, drumlins and the like—proglacial lakes, which are lakes formed either by the damming action of a moraine during the retreat of a melting glacier, or in the case of continental icecaps, by meltwater trapped against an ice sheet due to bedrock abrasion and major isostatic depression of the crust around the ice are highly visible indicators showing the maximum extent of the thick margins of northern continental glaciers. Look carefully at the following two satellite images noting that the white line marks the average periphery or terminus of the Each of the hemispheric ice sheets, and the white transparent region symbolizes the continental ices sheets themselves. The labeled lakes are terminal “proglacial lakes” formed along the margin of the ice sheet where not only maximum snow fall typically occurs, but also where maximum melting and scouring occur (because weather systems typically drop their moisture most heavily on the peripheries of ice sheets which warm and cold air masses meet).

A Few Definitions: Glacial Landforms
Before we dive into an explanation of proglacial lakes, lets quickly go over the definitions of a few important glacial features. GLACIER : A glacier is any persistent body of dense terrestrial ice which moves under its own weight. The term was created from the Old French “glace” or “ice” with a Savoy dialect ending likely denoting movement. Sea ice and lake ice are not glaciers. Neither is a thin ice layer under a thick snowpack that hasn’t started to move and plastically deform. Really, by definition a glacier needs ice thick enough to begin to shape the earth beneath it. ICE SHEET : An ice sheet (which is often used interchangeably with the word ‘Continental Glacier’) is more specifically an area of glacial ice that covers land (not sea) to an extent greater than 50,000 square kilometers (or 20,000 square miles). That’s roughly the size of West Virginia, Costa Rica or Bosnia. This somewhat arbitrary acreage was chosen in order to differentiate Ice Sheets from smaller Ice Caps or alpine glaciers. There are only two Continental “Ice Sheets” on earth—Greenland and Antarctica. ICE CAP : An ice cap is a bit of a misnomer and thus often wrongly used. It is not the ice which “caps” the the poles of the earth. It is the ice which “caps” a mountain and usually feeds a series of glaciers around its edges. Ice caps are smaller than 50,000 square miles. However, the term “polar ice cap”, referring to the Antarctic Ice Sheet, Greenland Ice Sheet and Arctic Sea Ice is used so frequently in the media that it is generally recognized as an acceptable use… even though it is technically incorrect. APLINE GLACIER : An alpine glacier or mountain glacier is a glacier which persists because of the effects of lower temperature with higher elevation and exists only within the constraints of a canyon, valley or topographic low. Alpine Glaciers often originate from mountain Ice Caps or even Ice Sheets. The peripheries of the Greenland Ice Sheet & Antarctica Ice Sheets are riddled with Alpine Glaciers exiting from mountainous canyons to the sea. However, its important to note that in the context of my articles, Alpine Glaciers are differentiated from the Continental Ice Sheets because without the mountains from which these glaciers originate, they would have never existed. This in contrast to the Continental Glaciers which form at or near sea level as a result of latitude instead of elevation. PROGLACIAL LAKE : A proglacial lake is a lake which forms at the downhill termination of a glacier. Proglacial lakes at the terminus of Alpine Glaciers are far smaller than the MASSIVE proglacial lakes formed at the terminus of Continental Ice Sheets. Proglacial lakes form in large depressions caused by glacial scouring. In massive ice sheets proglacial lakes often form as a result of isostatic depression from the weight of the ice. GLACIAL SCOUR SURFACE : When ice sheets move over relatively level surfaces, inconsistencies in the hardness of the bedrock create a distinct topography of lakes and linear erosional features called striations . In the case of Alpine Glaciers, these features can be small — ranging in size from a few centimeters to tens of meters. With Ice Sheets, however, these lakes and striations are often hundreds of meters or kilometers long and visible from space. Glacially scoured topography is one of the most obvious ways geologists know where a continental Ice Sheet existed. In places like the Canadian Shield or Scandinavian Shield the glaciers left a VERY distinct topography where nearly ALL topsoil and recent geological layers have been scoured away, leaving behind a distinct landscape of lakes, old bedrock and exposed linear features matching the movement of the ice mass. DRUMLIN : A long, low hill of sediments deposited by a glacier. Drumlins often occur in groups which are referred to as drumlin fields. The narrow end of each drumlin points in the direction of an advancing glacier. ESKER : A winding ridge of sand deposited by a stream of meltwater flowing underneath the retreating glacier. KETTLE LAKES : Lakes which form from chunks of ice left behind from the retreating glacier. GLACIAL PLUCKING : Plucking , also referred to as quarrying, is when a moving glacier exploits pockets of poorly consolidated bedrock or sediment, ‘plucking’ out a depression which later often forms a lake or pond in the substrate. Plucking is a process which helps to create distinctive Glacial Scour surfaces. THERMOKARST/PERMAFROST LAKE : Often confused with kettle lakes or glacial landforms, Thermokarst lakes ARE NOT glacially formed ! Also called a permafrost lake, thaw lake, tundra lake, thaw depression, or tundra pond, it is a body of freshwater, usually shallow, that is formed in a depression formed by thawing ice-rich permafrost in a tundra environment. They are very prevalent in Northern Alaska & Siberia. A key indicator of thermokarst lakes is the occurrence of excess ground ice with soils having an ice content greater than 30% by volume. They commonly form in ancient ‘oxbow lakes’ and deltaic deposits and form as pockets/aquifers of gravel freeze and thaw— both heaving/elevating the surface of area of greater groundwater content (causing surface erosion) and simultaneously collapsing the underlying substrate from the weight of the ice. (Chemical dissolution of underlying soils can also come into play–thus the ‘karst’) When this pocket of ice melts, a thermokarst or tundra/permafrost lake is left in its place. They are differentiated from glacial landforms by their shape, sediment composition and absence of any other accompanying glacial landform.
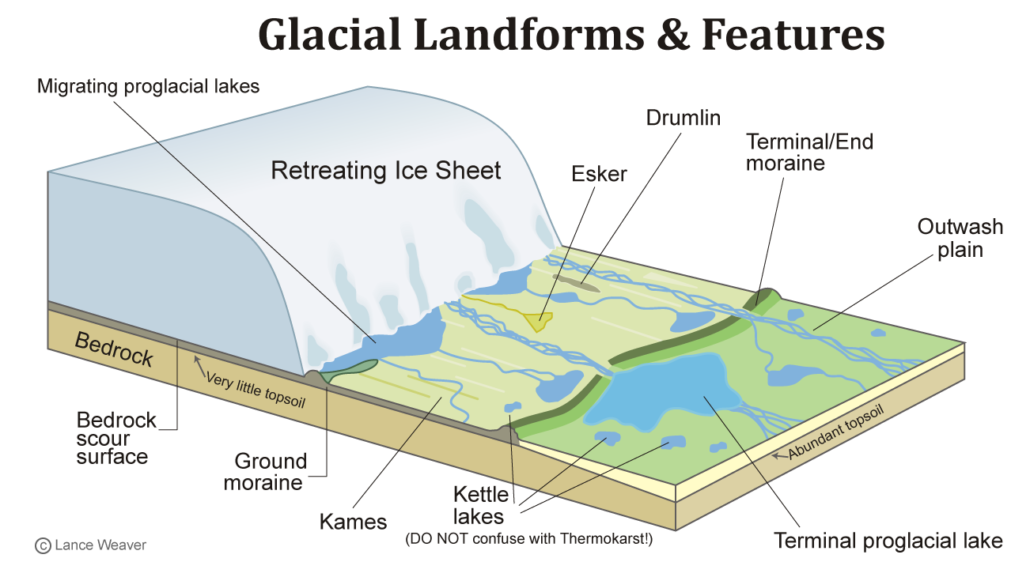
Once you understand the basics of these glacial features, its easy to simply use Google Earth to explore the regions shown above to make your own conclusions concerning the location of the Pleistocene Ice Sheets. I literally have not shared this with another individual who is even moderately trained in recognizing the preceding glacial landforms who has not come to the same conclusion that the location of Pleistocene Ice Sheets and absence of Glacial landforms in Northern Alaska and Siberia seems hard to explain without evoking some type of True Polar Wandering event. The difficulty and debate comes in when geologist try and decide, how on earth a True Polar Wandering event could have occurred in this short timeframe! These types of events become VERY debatable & problematic for professional scientists when attempting to use any type of catastrophism to explain. Why? I’ll explain in the next section, but first lets look at a satellite images to solidify in our minds the exact location of the Pleistocene ice sheets.
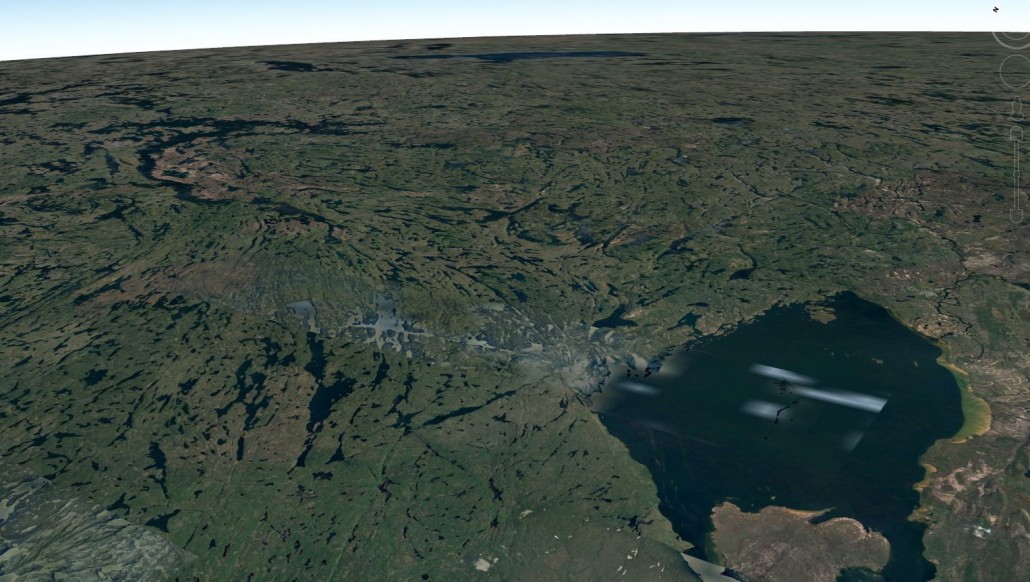
True Polar Wander as a Mechanism for Rapid Deglaciation
Both gradual and relatively rapid true polar wandering events are well established in paleomagnetic data throughout the geologic record. However, established methods used for dating the paleomagnetic evidence for these events has generally yielded time frames much greater than my hypothesized ~20 degrees of movement in >3000 years.
True polar wander is an known effect of non-symmetrical objects with multiple moments of inertia also known as intermediate axes. Because of the equatorial bulge and large mantle plumes , the mass distribution of the Earth is not spherically symmetric, and the Earth has three different moments of inertia axes. The axis around which the moment of inertia is greatest is closely aligned with the current rotation axis (the axis going through the geographic North and South Poles). A second axis is near the equator through the equatorial bulge. A third is theorized to also cross the equator at a right angle to the second, although mantle plumes and crustal imbalances from mountains or ice could cause it to locate elsewhere. However, if the moment of inertia around one of the two axes close to the equator becomes nearly equal to that around the polar/rotational axis, the constraint on the orientation of the object (the Earth) is relaxed. Even slight imbalances can make the Earth (both the crust and the mantle) slowly reorient until one of the second moments of inertia moves to the rotational axis or North Pole, with the axis of low moment of inertia being kept very near the equator.
This effect is explained in detail in the following Veritasium video on The Bizarre Behavior of Rotating Bodies
Paleomagnetic evidence for True Polar Wander is further explained in relation to paleomagnetic data in this video lecture by Dr. Trond Torsvik (CEED, University of Oslo, Norway) who has worked on paleomagnetics for nearly three decades.(TPW explanations start at minute 38:16)
Note that the above physics principles are remarkably similar to the Larmor Precession of atomic nuclides and might have something to do with the true relationship between true polar wander and the precession of the equinoxes as I explain a bit in my article ‘ Is the Orbit of Jupiter related to Solar Cycles ‘ .
As mentioned above, earth’s secondary moments of inertia are not only caused by the planets equatorial bulge but also large mass imbalances from plumes in the mantle seen by gravity anomalies in seismic readings. Many studies have been published mapping out and visualizing the structure, shape and location of these mantle plumes.
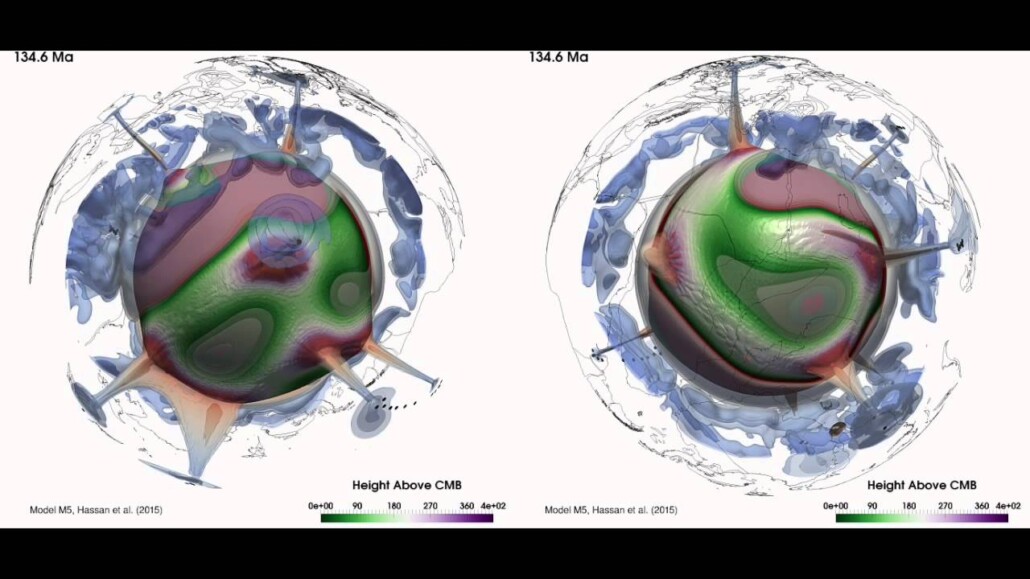
Nothing about True polar wandering, is controversial or unaccepted by geologists, geomorphologist and paleomagnetism researches in the mainstream scientific community. What IS controversial is rapid true polar wandering events spanning timeframes of less than a million years or so. Certainly my timeline of a few hundred to a few thousand years for a true polar wandering event will evoke debate. Toward the end of his life Albert Einstein appears to have been thoroughly convinced by many of Charles Hapgood’s arguments regarding rapid pole shift and perhaps even catastrophe. Einstein wrote in the foreword to Hapgood’s book , Earth’s Shifting Crust, “in a polar region there is continual deposition of ice, which is not symmetrically distributed about the pole. The earth’s rotation acts on these unsymmetrically deposited masses and produces centrifugal momentum that is transmitted to the rigid crust of the earth.” For Einstein and Hapgood, the very off-center weight of the northern ice sheet itself seemed a plausible mechanism to cause a rapid pole shift. Geologist (including myself), however, are not convinced. Not only did Hapgood’s brand of catastrophism seem too much like creationism, It simply did not explain the configuration of mid-oceanic spreading ridges, marginal continental subduction zones with their associated volcanic arcs and constant earthquakes, as well as evidence for the slow uplift of so many of earth mountain chains as well as Wagner’s Plate Tectonic Theory did. Nor does it consider the science of True Polar Wandering. Thus Hapgood’s theories were dismissed and discarded to await a future date when a new group of scientist more removed from the nineteenth and twentieth century passionate debates between uniformitarians and creationists, could relook at the evidences of Hapgood & Einstein and see if perhaps there was a combination of uniformitarianism and true polar wander that could explain the many arguments given in Hapgood’s work.
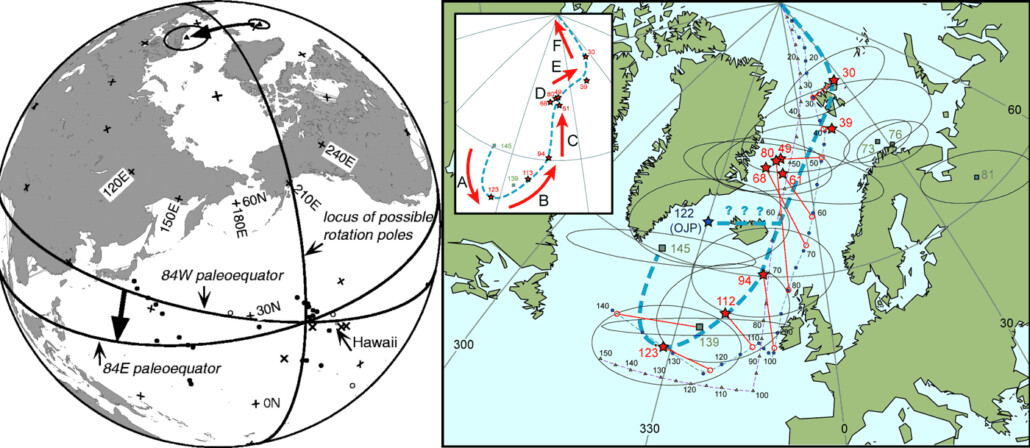
(Above. from The drift history of Adria and Africa from 280 Ma to Present, Jurassic true polar wander, and zonal climate control on Tethyan sedimentary facies Muttoni, et al, 2013 )
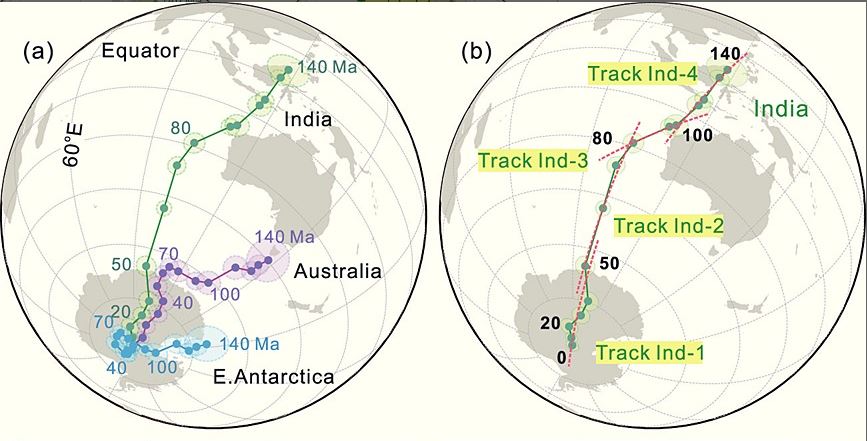
My illustration showing pole locations since the cretaceous.
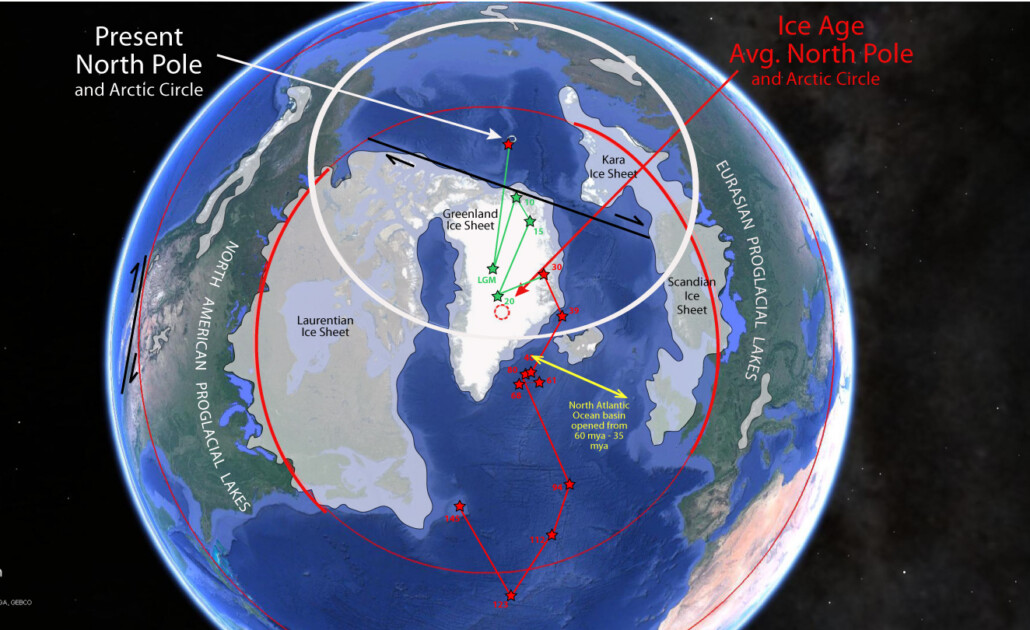
TO DO: Make a diagram here using the data from the above Sager pole location map, but showing it zig zag its way back and forth a bit better (in a processing 8 pattern like Atum rappelling apep in Egyptian book of Gates) with a proposed track all the way from parallel with England to the Pole.
-the arguments for uniformitarianism are multitudinous and convincing. From the astronomically controlled varves of many formations across the earth. To the timespans likely needed for evolution. To the river systems which are able to continue their way cutting deep passes into raising mountains.
-there are however many scattered instances of limited catastrophism as well though. Extinction events and the likely catastrophic comet impacts that caused them. Rapid climatic events like the younger dryas period and late Holocene climatic optimum /warming.
TO ADD/REWRITE INTO PAPER.
-Ice ages are nothing like we’ve supposed. They are not coming and going to any large degree. They began to form at the Eocene/Oligocene transition in BOTH the arctic and Antarctic when the Arctic circle was over Greenland (and possibly a few times before since the Cretaceous) and have persisted ever since with short ‘interglacial’. They were caused and ended by the location of the pole, which slowly wanders as a result of TPW driven by inertial forces caused by changes in polar precession. They began with the opening of the Atlantic when Greenland and Scandinavia separated in ~30 mya.
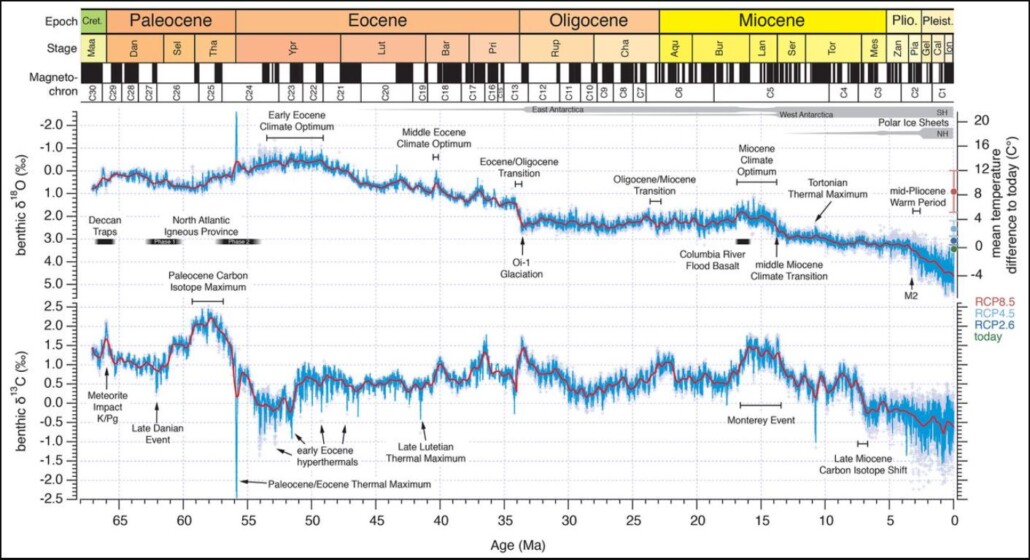
-Occilations for modern time periods also show JUST AS LARGE of delta/changes in oxygen isotope values. Easily showing 2% to 3% changes in isotope variances. See Knudsen et al, 2011 . Figure 4
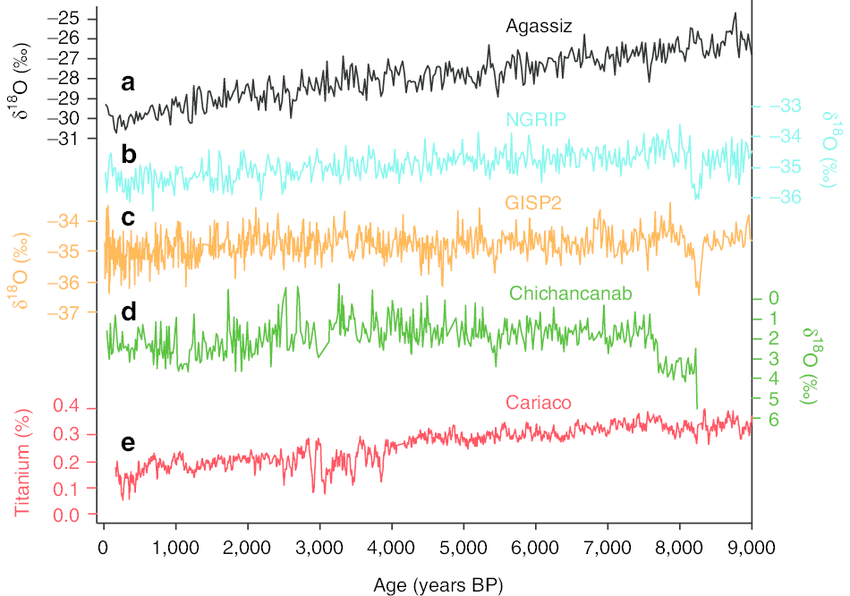
-Oxygen Isotope values CHANGE WITH SEASON & LATITUDE. That means that as the plates move and the pole drifts northward, the oxygen isotope values change slightly as well. Particularly as you approach the Arctic circle (as you can see from this article, Nakamura, et al, 2014 ) Note that studies like Hutchinson, et al, 2021 , show that Oxygen isotope values can vary from 1 to 2.5% depending on latitude. So when episodes of rapid True Polar Wander occur, it shows as an abrupt change in the oxygen isotope values (such as the Eocene/Oligocene Transition) [find an even better illustration with 6 or 7 locations at different latitudes]
-So How ON EARTH did we get convinced that 3-4% benthic oxygen18 isotope ratios means a glacial/interglacial? Look at figure-1 in this article (shown above). Glaciation in Antarctica started at 2%. If you look at the hot house/ ice house transition in the greater geologic record you can see that the benthic oxygen18 isotope ratios for glaciation/deglaciation in the Carboniferous/Permian to the Cretaceous varies from -2% to -5%, not 3 to 4%.
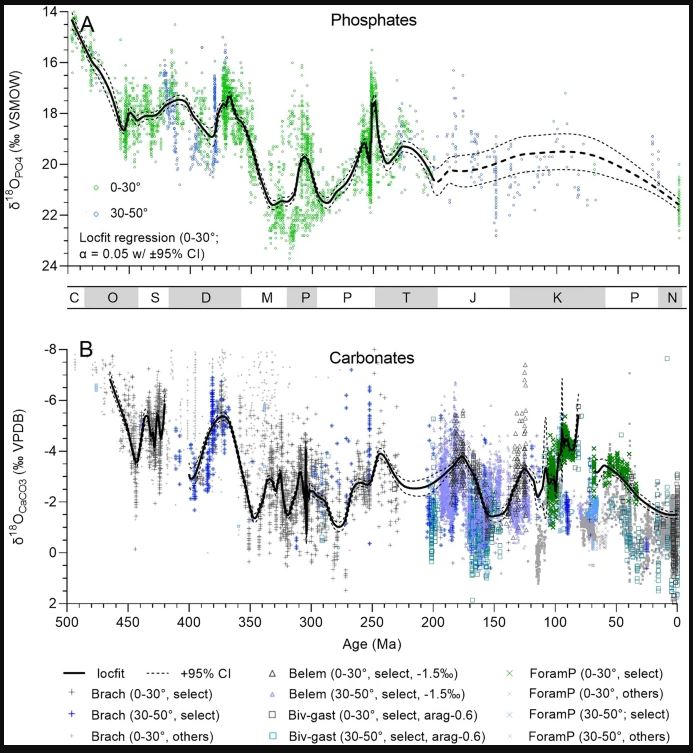
Overview: https://www.nature.com/articles/s41598-022-11493-1/figures/1 https://www.nature.com/articles/s41598-022-11493-1 Raw Data: https://www.researchgate.net/publication/331398373_Seawater_Temperature_and_Dissolved_Oxygen_over_the_Past_500_Million_Years/figures?lo=1 https://www.researchgate.net/publication/331398373_Seawater_Temperature_and_Dissolved_Oxygen_over_the_Past_500_Million_Years -This precession is the primary cause of plate movement and true polar wander -True polar wander is (define) -Its a lot like the X principle of a top, or the Y principle of an atom in an electric field. -The precession was likely begun and periodically altered by a combination of bolide impacts and electric field variations. -random bolide impacts and changes in the solar and galactic field strength change our processional amplitude and timing -The Oxygen isotope variations we see in Pliocene and Pleistocene have been misinterpreted at glacial cycles. They are not! The differences seen in pre-Pliocene vs post-Pliocene oxygen18 swings has to do with older/deeper material being compressed to a degree that flattens the curve. Show how summer/winter variations or secular variation like we’ve seen in the last 2000 years match the variation we see in the Pleistocene.
Compare these: https://www.nature.com/articles/s41598-022-11493-1 https://www.science.org/doi/10.1126/science.1221294 To these: !!!! (make an illustration of this!! https://www.sciencedirect.com/science/article/pii/S0277379122005613 https://www.sciencedirect.com/science/article/pii/S0277379122005613#fig5 https://www.ncbi.nlm.nih.gov/pmc/articles/PMC7614327/ (find a better one from more like 7000 BP to present. Or 20,000 BP to present.
TODO: Put the pole locations at different ages on this image as well as making an outline of North America and showing it move away from Europe and explain how ice age starts when the opening begins, and that the pole hasn’t drifted north hardly at all since 20mya, its just moved mostly west with North America. Show another location with the plate ages and more importantly, the Greenland Transverse Fault (which matches with the San Andréa’s Fault (starting 35-28 million years ago) but really accelerating with the swallowing of the Farallon plate/spreading ridge 20mya (draw spreading ridges on this image!)
-Add a graph showing the likely velocity of change for the pole shift. For instance, if it averaged 0.5 mph then it would take 2000 hours or 83 days to happen. I’m proposing something closer to 200 years. Which would be a speed of 0.00058 mph basically inperceptable.
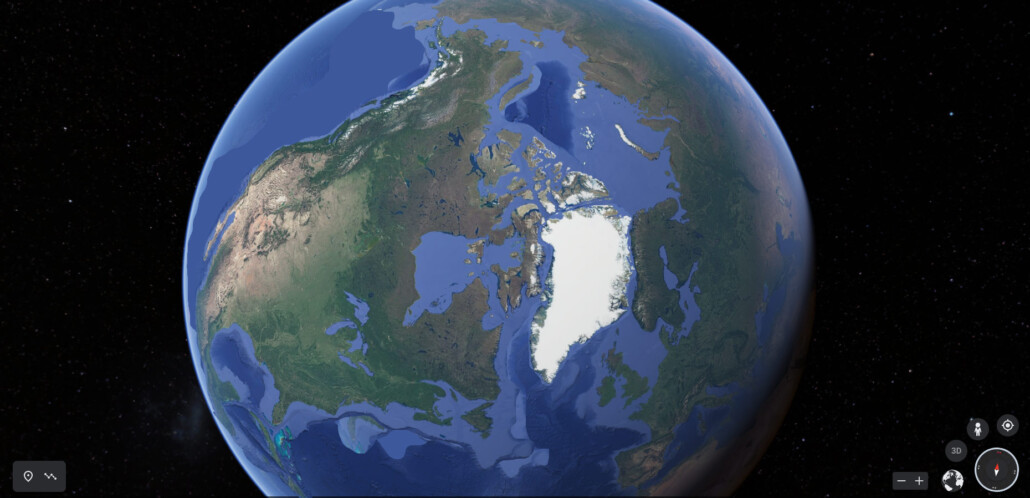
Thick Atmosphere Theory
This theory doesn’t hold up. First off, because dragonflies and bees would not have been able to evolve with their delicate wings in the Paleozoic like they did. Its more likely that pterosaurs had wings and feathers like modern birds. Although debated there seems to be more and more evidence of this being found. And they weren’t really that big. The biggest full fossil found had a wing span of 8.5 feet. Smaller than a modern condor or albatross. Must have been temp that changed the density of the mantle NOT an ancient thick atmosphere.
UNDER CONSTRUCTION FROM THIS POINT ON:
— add a sat image of Scandinavia scour surface. add a pic of thermokarst and how it is NOT a glacial landform.
NEXT section. Title: The Catastrophism/Uniformitarianism Debate . The debate is well documented. Until the early 1900’s it was the predominate explanation for earth processes. However, with time uniformitarianism won out. Why? Because it did a better job of explaining observed phenomena. However, because deeply held religious beliefs were often involved the debate had a tendency to polarize individuals into extreme camps . Instead of looking objectively at evidence and creating models based solely on logic, many individuals felt that had to take an “all or nothing” approach to their chosen camp. Now that decades have gone by, and people have had a chance to disconnect themselves from the emotion of this debate, a more rational middle ground is emerging. Somewhat catastrophic events such as the asteroid impact hypothesized to have caused the dinosaur extinction or to have caused the Younger Dryas cooling event during the Ice Age are becoming mainstream. Articles on ancient rapid true polar wandering are sprinkled throughout the scientific literature. I’ll finish this article when I have time. For now the rest is just notes.
TO DO: -most true polar wandering events proven in the literature occur on timetables of at least a million years. To rationally suggest a rotational axis change of 25 degrees latitude within just a few thousand years will require not only a believable mechanism, but physical calculations about how this could be accomplished without tearing the earth apart or causing overwhelming catastrophe with the changes in angular momentum & inertia. -talk about how this was a debate back in the 50’s and 60’s, especially with charles hapgood’s books (as well of velikovsky). But a believable mechanism for rapid pole shift was not conceived. Even though Einstein himself wrote the foreword to Hapgood’s book, and supported his theory that the weight of the ice itself destabilized the axis, the whole premise was too extreme, and smelled too much of biblical catastrophism for the scientific community to accept.
Talk about the comet impact theory for the Dryas Minima and how this combined with imbalances caused by the iceland mantle plume seem to some of the best explanations for a cause of the TPW
Paleomagnetic Reconstructions

Our current understanding of where pole is is based on several points of evidence….. POINT HERE NEEDS TO BE THAT RECONSTRUCTIONS ARE ONLY ACURATE TO 20 DEGREES, GIVES A GREAT BALLPARK FIGURE BUT HORRIBLE EXACT LOCATION. Be sure to reference this page, that shows how off CURRENT measurements are. http://all-geo.org/chris_rowan/2014/01/paleomagnetism-lab-testing-the-gad-hypothesis/
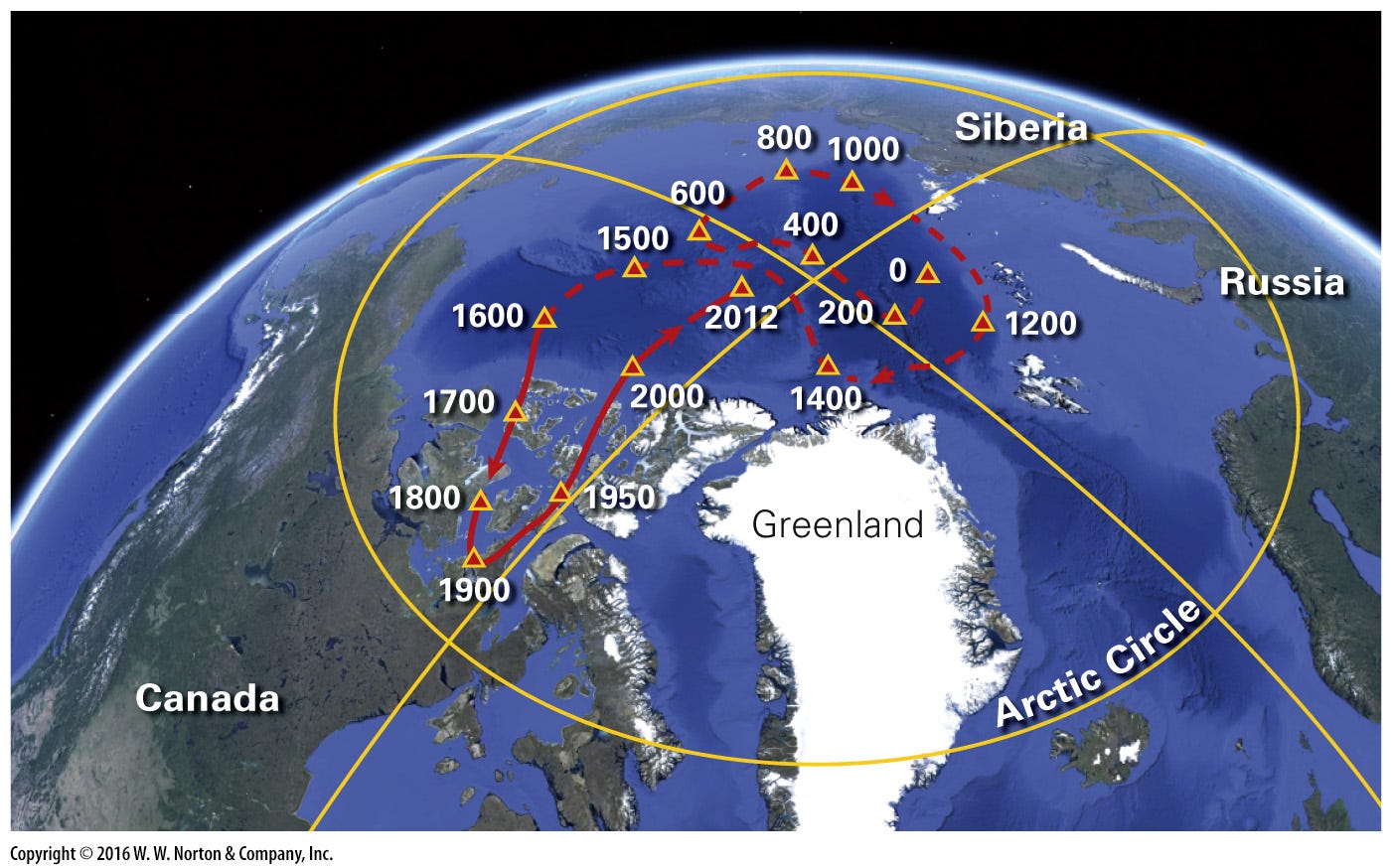
Possible Causes
Some are cyclical, some are random. Make an illustration showing the core slightly off center to show how earth would now wobble, and then stabilize over time.
- Gravitational effects of sun and Jupiter on moon and equatorial bulge (same things that cause precession-go into detail on how this is likely ALSO what creates the earth’s and suns magnetic fields, and same forces cause sun to flip and probably cause earth’s to flip too). There’s a good amount on the correlations between Jupiters 11 year orbit and the suns 11 year solar cycle (with Saturns gravitational effects causing the half year difference in timing). Note this important fact. The moon is NOT orbiting earth’s equatorial plane, its on the SUNS equatorial plane (2–5 deg off), so the combined tidal forces of the moon and sun pull earth’s core double and unevenly .
- Bolide/asteroid impacts. (this is a big one, partly random and partly cyclical because arms of galaxy hold more debris. The proposed impact for the younger dryas is a great possibility as one of many)
- Gravitational waves (also a big one, because it literally warps space time and compresses the earth longitudinally. can be both cyclical and random between those coming from center of galaxy and those caused by supernova). Reference my article on how these are responsible for the arms of our Galaxy, and how when we cross these waves every 4000 years or so, it cause slight wobble events. (which if there is enough built up instability triggers a TPW event).
-TPW “excursions” caused by “destabilization events”. The evidence for glaciation in Patagonia and Siberia is a huge problem. It seems to suggest that the pole must have gone from north of present to greenland and back! How is that possible? And it doesn’t match well with any polar wandering data (of course those kinds of anomolous data points are often thrown out of studies). And why is Antarctica’s glaciation so much older than the north hemisphere? South-East Antartica should have the oldest ice. North-East Antartica & the Penensula should have the youngest. Does this match with data? See evidence for each area’s glaciation here: http://www.science.earthjay.com/instruction/HSU/2015_fall/GEOL_553/literature_sources/ehlers_gibbard_2007_cenozoic_global_glaciation.pdf — — -this what you have to work with. So this idea solves it. THE CORE is more dense, it gets pulled by gravity more and destabilizes the earth. BUT the equatorial bulge resists the dif spin, causing mantle to differentially rotate (making the mag field by the way). but every 100,000 radiocarbon years or so the destabilization hits a tipping point and the SLOW TPW looses balance and the earth goes for a TPW trip. For the last 3 million K/Ar years this has been TOWARD the “North Atlantic gravity anomaly” (there also one north of Australia likely playing a part). And asteroid hitting the moon might just knock its orbit eccentric enough to affect tidal forces on the mantle.
Evidence Against Catastrophism
Be sure to add a whole section here on what I see in river systems and orogenic uplifting and folding on the Colorado Plateau. Its important to see the powerful evidence for uniformitarian orogenesis in earth’s river systems which traverse folded strata. For instance, even the smallest tributaries of the Colorado River cut through massive folds which obviously have risen slow enough over their evolution to avoid rerouting of the river systems. Only a few examples such as the Unaweap Canyon through the Uncompahgre Plateau show evidence of rapid enough uplift to reroute the river. This suggests to me that although these pole shirts are occurring fast in “geologic time”, they are not occurring fast enough to catastrophically reorganize plate motions.
Now this is where it gets crazy
Rapid polar wander events are fairly well documented and accepted in the Scientific literature. Just one of many examples is the Jan 2000 issue of Science which published evidence of “ Late Cretaceous Polar Wander of the Pacific Plate: Evidence of a Rapid True Polar Wander Event “. These types of events are typically hypothesized to occur over about a million years. And of course debate will always exist as to whether these perceived rapid polar wandering events are simply movement of the magnetic pole , instead of true polar wandering of the rotational pole or axis (since in the geologic record it is difficult to tell the difference between the two). Debate also rages as to which reference system is the most reliable, as every continent and seamount system gives a different APW (apparent polar wandering) path since the plates all move separately from each other over time. However, to me the most interesting data point is the lack of Paleozoic strata under the entirety of the Pleistocene polar ice sheet! Where did it go? Are we to believe as some suggest that the entirety of the Canadian & Scandian Shields were above sea level for the last 500 million years in a configuration matching exactly with the ice sheet. That seems entirely impossible to me. Or are we to believe that the Pleistocene Ice Sheet removed/eroded essentially ALL Paleozoic strata? Although far more likely than the first possibility, this still seem unlikely to me. So then where did it go? When I see that the seamount reference frame shows an APW (apparent polar wandering) path matching closely with the location of the radiocarbon dated Pleistocene Ice Sheet it simply seems more likely to me that some type of ice sheet actually existed in that area for far longer than the Pleistocene. Perhaps more likely since the Eocene thermal maximum, when the Antarctic ice sheet is known to have began growing (or possible even into the supposedly “hot house” Cretaceous). Looking at the data, I cant get past the most obvious answer, that a Scandian & Canadian Ice Sheet existed in these “ice age” locations for much of the past 45-120 million years, and that this explains why there is essentially ZERO Paleozoic sediment in those locations. And that some kind of mechanism (likely a mantle plume) actually has had the rotational axis shifting back and forth from at least the end Eocene — causing along with it some type of overlap in radiometric dates. (
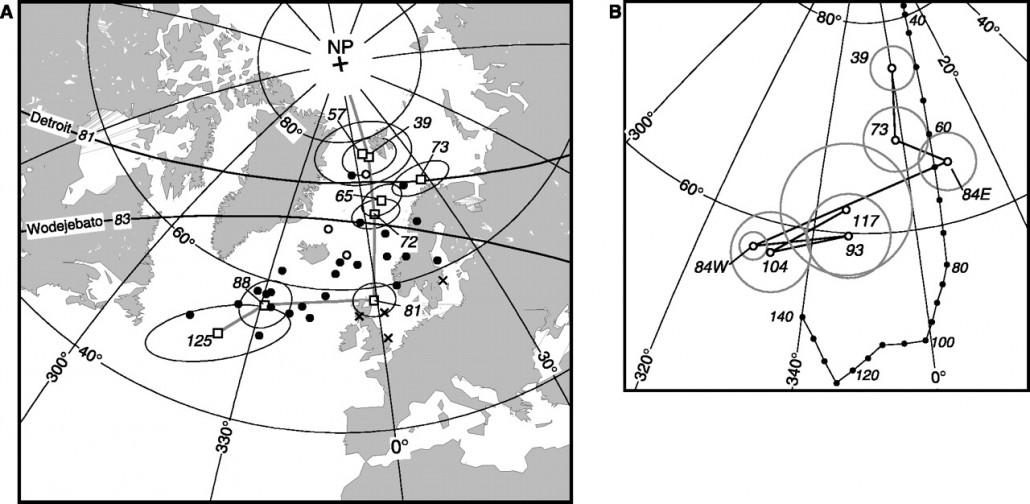
Unraveling the mystery. A few other really significant pieces of evidence which all seem to come together & support not only rapid true polar wander, but a large enough climatological shift to affect radiocarbon (likely from a changing magnetic field) 1. The fact that the rise of known civilization occurs completely counter to what modern climate suggest we should see. Modern farming productivity and thus population and settlement is NOTHING like that of the ancient world. Why did civilization rise in Sumer/Akad, Egypt and the Middle East, when modern climates make these HORRIBLE places to grow civilizations?! (compared to the Modern age population centers of Europe, China & the Eastern US.) All sorts of theories abound, but all of them are lacking in common sense. Yet when you look at the latitude lines from the last “Pleistocene Ice Age”, Egypt and the Middle East would have been the “Europe” or temperate region of the ancient world! (30-50 deg latitude). In fact comparing the amount of climate change which would have occurred in different regions of the world corresponds beautifully with where ancient civilizations persisted. Southern Africa & lowland S. America for instance would have suffered enormous devastating climate swings which might explain why no major ancient civilizations seemed to have evolved in those locations. On the other hand the climate change in places like Anatolia, and the Iranian Plateau would have been far more minimal. Which perhaps explains why this is where we find some of the most ancient cities in the world. 2. Testing this hypothesis we would of course expect to find evidence of radiocarbon anomalies, and this is perhaps the most convincing evidence I see of a TPW event. There is a very strange ‘repeat’ of history seen in the progression of Middle Eastern civilization. (make a bunch of illustrations and timelines of the repeat between radiocarbon dated civilizations— and how crazy obvious it is once you see it.)
Notes And References
– A significant issue in suggesting smaller time frames to the Last Glacial Maximum lies in the time needed for reforestation of the region. In this article, pollen samples’ suggest trees existed in small pockets or refugia throughout glacial maxima periods. .Laura Parducci, et al. Glacial Survival of Boreal Trees in Northern Scandinavia, Science 335, 1083 (2012) https://www.researchgate.net/publication/221882445_Glacial_Survival_of_Boreal_Trees_in_Northern_Scandinavia
-The DATED-1 glacial eurasian database. Probably the best source of exact eruasian ice boundaries available. Hughes, Anna, Gyllencreutz, Richard, et al. The last Eurasian ice sheets – a chronological database and time-slice reconstruction, DATED-1. Boreas Volume 45, Issue1. January 2016. Pages 1-45. 2015 h ttps://onlinelibrary.wiley.com/doi/full/10.1111/bor.12142
– Eurasian glacial reconstructions going back to around 140ka John Inge Svendsen, Helena Alexanderson, et al. Late Quaternary ice sheet history of Northern Eurasia. January 2004Quaternary Science Reviews DOI: 10.1016/j.quascirev.2003.12.008 https://www.researchgate.net/publication/223294900_Late_Quaternary_ice_sheet_history_of_Northern_Eurasia
– Illustration from video of circular plate motion vectors going around an equatorial secondary axis from True Polar Wandering. Steinberger, B. Torsvik, T. Absolute plate Motions and True Polar Wander in the Absense of Hotspot Tracks. Vol 452| 3 April 2008| doi:10.1038/nature06824 https://www.researchgate.net/profile/Bernhard-Steinberger/publication/5466242_Absolute_plate_motions_and_true_polar_wander_in_the_absence_of_hotspot_tracks/links/0fcfd5122024b06d8e000000/Absolute-plate-motions-and-true-polar-wander-in-the-absence-of-hotspot-tracks.pdf
– Evidence from 40 Ar/ 39 Ar dates suggest a rapid wander event of 3 to 10 degrees per million years during the Late Jurassic/Early Tertiary. (see counterargument ) William Sager & Anthony Kopper. ‘Late Cretaceous Polar Wander of the Pacific Plate: Evidence of a Rapid True Polar Wander Event .’ Science Magazine . Jan 21, 2000, Vol 287, Issue 5452, pp. 455-459. DOI: 10.1126/science.287.5452.45 https://www.researchgate.net/publication/223779271_A_Late_Cretaceous_pole_for_the_Pacific_plate_Implications_for_apparent_and_true_polar_wander_and_the_drift_of_hotspots – Paleomagnetic study using the Hawaii hotspot as a fixed reference point, this paleomagnetic study showed the North Pole moving within the Greenland and North Sea region since at least the Late Cretaceous. William Sager. ‘Divergence Between Paleomagnetic and Hotspot Model Predicted Polar Wander for the Pacific Plate with Implications for Hotspot Fixity’. University of Houston, Draft 23 November 2006. Draft here . Published in “Plates, Plumes and Planetery Processses”, found here . http://www.mantleplumes.org/P%5E4/P%5E4Chapters/SagerP4AcceptedMS.pdf
-11 year magnetic reversal of the sun tied to orbits of Jupiter, earth and (venus?) in new study https://www.eurekalert.org/pub_releases/2019-05/hd-tsf052719.php
Best article to cite. Lots of great references. Study it! https://www.sciencedirect.com/science/article/pii/S0012821X1630749X?fbclid=IwAR1Y-bgol8Sd1IzmsfcsFALQ_2xzY_dnrHXnR8CZAJTTEOuAN2V2TeqQiuI#br0170
-This page has some amazing gifs, illustrations, videos and ideas to use. especially the gif of an egg shaped spinning object righting itself, and the evidence of why Venus turned over. and some of the other gyroscope stuff. https://www.plutorules.com/page-41-tilt.html This similar page talks of how ‘rain forest like in new Zealand covered Antarctica at the same time https://www.bbc.com/news/science-environment-12378934
-this wikipedia article talks about how the ‘poles’ were dominated by ‘ deciduous conifers’. whaaat? Conifers like Larch and Cyprus that loose their leaves and live in wet , not-too-cold enviros like Washington state. Its yet more evidence that what they think was artic, was not artic. https://en.wikipedia.org/wiki/Polar_forests_of_the_Cretaceous#Fossilized_forest
-Evidence against current magnetic polar paths . This dinosaur is found in Australia and Antartica in the early cretaceous. scientist think this was in the antarctic circle at the time based on paleomag. This is great proof the paleomag is wrong. https://en.wikipedia.org/wiki/Leaellynasaura
-Younger dryas north hemisphere cooling (mainly europe) didn’t affect new zealand. Glaciers there were retreating with vigo https://www.sciencemag.org/news/2010/09/deep-freeze-didnt-affect-southern-hemisphere . but Radiocarbon dating of this time interval is precarious because of C14 plateaux and, for marine organisms , because of the varying reservoir effects as a consequence of changing ocean circulation (Björck, 2007; Cao et al . , 2007). https://www.sciencedirect.com/topics/earth-and-planetary-sciences/younger-dryas (looks to me that they see the younger dryas is regional, so they are guessing the c14 dates must be off and coming up with this ‘reservoir effects’ idea where they compensate…
-evidence for comet impact at younger dryas, this is a great mechanism for what made the pole change direction at that time.. https://wattsupwiththat.com/2012/09/20/support-comet-impact-may-have-triggered-the-younger-dryas-period/
Malankavitch cycle… one of three is ‘precession’ and might cause true polar wander because of gravitational forces of sun and Jupiter. (especially since they are binary system). The moon rotates obliquely so that affects things too. watch this and picture the sun torqueing irregularities in the mantle and equatorial bulge. https://www.youtube.com/watch?v=ty9QSiVC2g0 see also Larmor Precession .
rework malankavitch calcs to show it could NOT give needed cooling, then propose mantle redistribution combined with gravitational pull from sun/Jupiter wobble (chandler wobble) to create true polar wandering as mechanism for both ice ages and plate movement.
younger dryas (north america warming, Bonneville shrinking while Europe cooling lgm)
Explore this: Even in more ancient geologic record, paleomagnetic reconstructions show that at least the magnetic pole (and likely true north pole) is all over the place through time. Comparing magnetic trace readings from different continents and oceanic plates show paths and sudden swings which cannot be accounted for with plate techtonics. These paths suggest that not only are the plates moving and separating over time, but the magnetic north pole (and presumably the true north pole with it) is moving. If we are to assume that the magnetic pole has historically been within 10–13 deg of the true north pole, we
WOW OMG…I have always been fascinated by glaciers, and back in 1974 when I should have graduated with a degree in Geology and wanting to discover and prove that Idaho still had a small active mountain glacier! Somebody beat me two it, on Borah Peak; although there are possibly two more in the Pioneer Mountains!
Your article makes sense! IT JUST PLAIN MAKES SENSE! Someway, somehow the geographical North Pole wandered…some kind of catastrophic event…comet, meteor, massive solar flare….very obvious signs of advanced civilization worldwide!! megaliths structures) capable of carving / molding / moving thousand ton blocks with micrometer precision! Modern science unable to explain or duplicate…WIPED OUT 10s of thousands of years ago!! I have ya bookmarked …your article, an amazing insight…I cannot unsee it because it just makes sense 🤔🤔🤔
This is not a mystery of the ancient past but of modern man “The Pole Shift that Sank Atlantis”
Derek, you are more right than you imagine. Plato was not overimaginative about Atlantis. About 20 years ago a German scientist found it. And it was in a very vulnerable location. This article is on the right track, but it gets particulars wrong. One big question is why Earth had an ice cap that was not at the pole.
If you would like to take a look at my website jfalthouse.com . I have been working the same problem from a different direction. I spent the last 40 years documenting the magnetic orientation of about a hundred ancient sites in the Americas, Europe, and Africa. I noticed that monuments built about the same time, orient towards the magnetic north pole where it was positioned at the time of that construction. Even better, multiple sites triangulate on a particular magnetic north pole position from sites of the same age, spaced thousands of miles apart.
I like Wesley Patten’s theory on what caused the ice caps to form, vanish, and re-form over the eons. My research seems to agree with his belief that the ice caps were created each time via an ice dump from space, two to five miles deep of ice in about six hours.
jack althouse RA NCARB
So, The crazy thing that my Geology professors tried to convince me of is that there was an ice sheet that covered as far south as Connecticut, but didn’t TOUCH Alaska! What?
I don’t buy it. You seem to be overthinking it. For a glacier to grow, it just takes a net gain of snow in a year. And for an ice sheet/glacier to remain it just needs to break even with snow gained vs lost. The Ice Age glaciers of North America and NW Europe were made possible by substantial winter precipitation AND low summer temperatures. But the low summer temperatures followed the snow buildup as opposed to the other way around. When you get a massive ice sheet pushing south, it manipulates the climate immediately south of it so that it becomes much colder. When moisture-laden winds blow in, the result is a large amount of snow dumped at the edge of the glacier, causing it to expand further and the process repeats.
This is important because eastern North America is bound by warm ocean currents in the Atlantic and Gulf of Mexico which bring warm moist air deep into the continent. It’s the same in NW Europe and the northern Pacific coast of Canada/US, all areas that had massive continental ice sheets. Warm moist air flowing into the outer boundaries of ice sheets during winter is necessary for the growth of these glaciers during Ice Ages.
And by the way, Kamchatka and southern Alaska WERE heavily glaciated back then and still are to a lesser extent now.
And why not Siberia? It was drier all year round compared to now but similar to now, the aridity was greatest during winter. And since these winters were so dry, no major snowpack built up by the time summer rolled around and it all melted. No snowpack=no large ice sheets in the north that could manipulate the climate and reduce summer temperatures further south=a vast unglaciated area that was a steppe-tundra. If Siberia and by extension East Asia were exposed to wetter winters, you can almost guarantee there would’ve been a large ice sheet extending into northern China and perhaps even Korea.
Long story short: Cool summers, lots of snow in winter(Kamchatka/southern Alaska mountains)=Good for glaciers to form Cool summers, small amount of snow in winter(central Antarctica)=Ok for glaciers to remain Relatively warm summers, lots of snow in winter(Adirondack mountains, northern Japan)=Bad for glaciers to form Relatively warm summers, very little snow in winter(Siberia)=Terrible for glaciers to form
I get how when you see something one way or are taught something your whole life its hard to change your thinking. I’ve been studying the arguments you give most of my life, and they really just don’t add up. They’re certainly possible. But just not very logical.
In my opinion, its these ‘microclimate’ arguments you are making that are over thinking it. But I address why these are so poor in the article. Hop on my app, and explore the areas and logic about what your saying in relation to both Alaska and EASTERN Siberia. Both these regions have warm moisture-rich currents which bring phenomenal amounts of precipitation into the regions. Yet neither of these regions had anything but mountain glaciers. Your logic on which areas were glaciated and which weren’t is entirely circular (even if only slightly more circular than the actual shape of the lopsided Pleistocene continental glacier ;)
We know where ice was and wasn’t so you just say “conditions were NOT right in north Japan or Manchuria…”, but if we knew there had been a non-mountain continental glacier there. You’d just say… “conditions WERE right.” But when you look at annual snow accumulation in those regions, you see how poor that circular reasoning is. A predictive model which didn’t already know the answer it wanted would NOT come to the same conclusions. Even central Siberia gets more snow than central Antarctica. Northern & central Alaska gets FAR, FAR more snow. Northeast Manchuria too. Yet none of these regions had substantial non-mountain glaciers and the only reasoning that is employed to explain that is circular reasoning.
Aaaaand, much like modern Greenland, and unlike New York, Britain or Scandinavia, regions like central Alaska or eastern Siberia have MASSIVE mountain accumulations zones which can serve as nucleation spots for the “glaciers create more glaciers” microclimate argument. But once again… they had NO substantial non-mountain glaciation during the entirely of the Pleistocene.
If New York and Maine had thousands of feet of ice extending down to sea level, reshaping Long Island and Cape Cod, then surely North Yukon, East Siberia, B.C., Oregon and all the way down to northern California should have too! But instead, the glaciers in those regions stayed up in the mountains and canyons and really didn’t substantially reshape terrain outside of the lower canyon lobes and high elevations. With your reasoning the entire north Sacramento valley should have been one big glacier, leaving substantial landform modifications. I mean SURELY, the cascades (which are north of the Laurentian south lobes) would have created a microclimate which made a truly MASSIVE west coast glacier bigger than the Adirondacks. But no.
This does not appear to be overthinking at all. The shields evidence is quite intriguing as are the positions of the proglacial lakes. I wouldn’t get hung up on the “how” regarding geoshifting of the poles, but more importantly keep focused on what the ice did and where it came from/went. It looks like there are competing thoughts on what was and was not continentally glaciated. That should not be hard to get past. There is no eastern Russian / Kamchatka / Siberian shield. Super odd… If indeed as you claim the north pole was somewhere over Greenland, then your thinking is validated again by the conditions in Antarctica. Greenland is antipodal to eastern Antarctica, but the geoshifting certainly would have had an impact on New Zealand and Southern Austraila? Look into that. Of course what no one mentions is that exposed landmass was nothing like it is today during the last glacial maximum. In fact sea level was 400 feet lower. Huge strike against the microclimate comments. Both the Alaskan and Siberian landmasses were far larger (and connected). Australia was also a much larger landmass – exposed land connected Indonesia and Australia. Simply stated, microclimates were vastly different with greater exposed mass of land. Given that continental glaciation reached as far south as Kansas, I have often thought that Hudson Bay was the geographic north pole. Lastly – back to the shield discussion. At least one member of the Royal Geographical Society was discredited for claiming he had crossed the arctic circle in Northern Canada based on the observation of taiga above the circle in both Alaska and Northwestern Canada (of course in Siberia as well). It would make no sense to me either, but given that the old location of the pole created shield conditions this was actually factual observation of taiga above the circle, but also explains arctic tundra as far south as the southern extent of Hudson Bay. That would make absolutely no sense if the geographic pole was where it is now. So, please take a look at where arctic tundra / taiga is amassed in Canada.
Guest – Like climate, ice is not a causative agent. It is the tail end of other causes. And ice does not – cannot – move on flat ground more than a handful of kilometers. The academics have got nearly all of it wrong.
Lance: Just superb graphics. Excellent work. Very hard to do. As you likely know there have been a number of others who have used varying methods to determine the last – or even previous – poles. Many normal Geologists started with the theories in the 50’s and you can tell their starting source by where they say (the last one) was: Hudson Bay/Hapgood, Baffin Island/Velikovsky. Since then, a number of others have used various “evidences” to fine tune the exact locations, timings of, and whether they were sudden or slow wandering. Also causation, but that has been a stumper. I have most of their work, and my own “cause”, which differs. I can clearly understand why you blocked your email. Too many “Nattering Nabobs of Negativism” to try to delve into this most important mystery with wide-ranging open-minded thinking. I give you my email and then later perhaps my website if you want to start a discussion. I have not fully digested this piece of yours. That is my next task. Thank you for the work you have done. KW
This is quite impressive and graphics very clearly presented. The idea that the Earth’s Pole may have shifted is not new. In about the 1680’s Edmund Halley suggested that the North Pole could have been in or near Northern Greenland. Halley had spent time as a ships Captain plotting magnetic anomalies in the North Atlantic and noticed that the magnetic North Pole was as near to Greenland and its substantial ice fields as to the North Pole. He suggested an impact with a comet or asteroid might have substantially shifted the Earth’s geographical Poles. The distribution of the great mass of ice in the last Ice Age does appear weird. Canada and the northern States of USA glaciated but parts of Alaska ice free together with much of Siberia. Your animated graphics linked to Winter and Summer present locations for the northern extent of snow and ice show how closely they relate to their latitude. More food for thought as they say.
Fascinating idea, which would explain many palaeoclimatic observations in a simple way without resorting to the many over-complications that are adopted in palaeoclimatic models to explain sudden geographical variations. Here the scientific problem would be to investigate the physical mechanism that could explain a super fast true polar wander. Let me add a recent article from science, https://www.science.org/doi/10.1126/science.ade4248 ,the smoking gun?
Have you or anyone else done any additional research on this? I’m fascinated, because it ties in with another project I’m working on. It focuses on the fairly dramatic ice cover melt in West Antarctica circa 10,000 to 12,000 BP. ( https://www.nationalgeographic.com/science/article/west-antarctic-ice-sheet-collapse-climate-change ) What’s fascinating is if you plot a new South Pole based on your proposal, it puts West Antarctica fairly far outside the Antarctic Circle, and might explain the interesting results found in the NatGeo article.
Yes, I’ve been following that research with interest. I’ve been working on a book which combines all the ideas in this article with the evolution of the Colorado River, and I plan on adding the new Antarctic Peninsula data. Are you working on it in an academic setting?
Leave a Reply
Leave a reply cancel reply.
Your email address will not be published. Required fields are marked *
Latest Blog Posts

Important Pages
Relevant links.
- Utah Geological Survey
- U.S. Geological Survey
- Arizona Geology Blog
- Durango Bill
- Geology.com


IMAGES
COMMENTS
Polar wandering, the migration of the magnetic poles over Earth's surface through geologic time. Although research began in the early 1900s, it was not until the 1950s that data suggested that the poles had moved in a systematic way. Polar wandering research has provided evidence for the concept of continental drift.
Polar wander Polar wander is the motion of a pole in relation to some reference frame. It can be used, for example, to measure the degree to which Earth's magnetic poles have been observed to move relative to the Earth's rotation axis. It is also possible to use continents as reference and observe the relative motion of the magnetic pole relative to the different continents; by doing so, the ...
Following theoretical work on 'polar wandering' in the late nineteenth century, Wegener (1929) (English translation by Biram (1966, pp. 158-163)) recognized that continents might appear to drift not only at the behest of spatially varying internal forces but also by steady or punctuated whole-scale shifting of the solid Earth's reference ...
True polar wander: A major shift The Tokyo Institute of Technology said on October 18, 2021, that one of its researchers helped uncover new evidence for what's called true polar wander.
Continental drift, once a theory on the fringe of the scientific community, is now a well-established phenomenon. The idea that continents move around on the surface of the Earth has been supported by overwhelming evidence from many different sources. Polar wondering as evidence of continental drift is now a widely known fact. The method of polar wandering uses magnetic data to track how the ...
True polar wander. True polar wander is a solid-body rotation of a planet or moon with respect to its spin axis, causing the geographic locations of the North and South Poles to change, or "wander". In a stable state, the largest moments of inertia axis is aligned with the spin axis, with the smaller two moment of inertia axes lying in the ...
True polar wander. True polar wander is a solid-body rotation of a planet or moon with respect to its spin axis, causing the geographic locations of the north and south poles to change, or "wander". Unless the body is totally rigid (which the Earth is not), its stable state rotation has the largest moment of inertia axis aligned with the spin ...
Earth is undergoing true polar wander, scientists say. In late 2012, scientists based in Germany and Norway published new results about a geophysical theory known as true polar wander. That is a ...
Plotting the apparent polar positions for rocks of different ages from North America and Eurasia produces two curves, the so-called "polar wandering curves". Note that as the curves get younger they converge. Fitting the continents back together results in a single curve.
What has eluded researchers is a theory that clearly explains how and why the pole returns to its original location, or the "oscillatory true polar wander". In the new work, graduate student Jessica Creveling, also of the Earth and Planetary Science Department at Harvard, along with Mitrovica and colleagues, provides an explanation.
It appliesequally to polar wandering, as emphasizedby its following corollary: If a once rigid body, initially in pure rotation about its principalaxis with the largestof three unequal moments of inertia, were transformedgradually into a new rigid shape by internal processes alone, its subsequentrotation axis would still coincide almost exactly ...
Earth's outer layers can wander over its core, which causes so-called true polar wander. A model has figured out how Earth's bulge may cause the layers to snap back into place.
Scientists explain magnetic pole's wanderings. The North Magnetic Pole has moved rapidly in recent years away from Canada towards Russia. European scientists think they can now describe with ...
Palaeomagnetic data from South China and compiled reliable palaeopoles from 4 other continents reveals a ~50˚ true polar wander (TPW) event occurring 450-440 million years ago. Sweeping ...
Polar wandering (similar to Continental Drift Theory) Polar wandering is the relative movement of the earth's crust and upper mantle with respect to the rotational poles of the earth.
The models show a ridge (a) about 5 million years ago (b) about 2 million years ago and (c) in the present. [1] Paleomagnetism (occasionally palaeomagnetism) is the study of prehistoric Earth's magnetic fields recorded in rocks, sediment, or archeological materials. Geophysicists who specialize in paleomagnetism are called paleomagnetists.
The ancient rocky lurch was part of a phenomenon known as true polar wander, in which the topmost layers of the planet, likely all the way down to the liquid outer core, rotate significantly even ...
Earth's geographic poles may seem like immovable fixtures, but new evidence suggests that they weren't always where they are now. The findings support an old but controversial theory known as "true polar wander" that states that the poles moved as part of a planetary balancing act millions of years ago.
Abstract The rate of movement of Earth's solid shell relative to its spin axis, or true polar wander, depends on variations in mantle convection and viscosity. We report paleomagnetic and geochronologic data from South China that constrain the rate of rapid true polar wander (>5° per million years) between 832 million years and 821 million years ago. Analysis of the paleomagnetic database ...
True polar wander is an known effect of non-symmetrical objects with multiple moments of inertia also known as intermediate axes. Because of the equatorial bulge and large mantle plumes, the mass distribution of the Earth is not spherically symmetric, and the Earth has three different moments of inertia axes.
The magnetic polar wandering effect, also known as apparent polar wander, refers to the phenomenon where the magnetic poles of the Earth appear to move relative to the continents over geological time. This effect is crucial for understanding plate tectonics and the history of the Earth's magnetic field. Here's a detailed explanation of the ...
Apparent polar wander Apparent polar wander ( APW) is the perceived movement of the Earth 's paleomagnetic poles relative to a continent while regarding the continent being studied as fixed in position. [1] It is frequently displayed on the present latitude-longitude map as a path connecting the locations of geomagnetic poles, inferred at distinct times using paleomagnetic techniques.
Polar wander and water ice The movements of the moon's poles also have another significant effect.
Polar bears spend their lives wandering the Arctic sea-ice that has no delineated borders. The beautiful lumbering beasts can meander—20 to 50 miles a day and hundreds of miles in a year—across countries and even continents in the frozen region circumventing the North Pole. They've been tracked moving across the ice from Greenland to Canada and back, which is one reason scientists are ...